Biomolecular evaluation of three contrasting rice cultivars (Oryza sativa L.) in salt stress response at seedling stage.
DOI:
https://doi.org/10.14719/pst.1539Keywords:
ROS, Quantitative real-time PCR, Rice (Oryza sativa L.), Isozyme, Salt tolerance, Na -transporter geneAbstract
Salt contamination of soils due to climate change faces a severe environmental issue that affects crop production today. However, the response mechanism in plants to salt stress is not fully understood. The present study investigated molecular and biochemical changes under salt stress in rice seedlings of three rice cultivars, i.e., AGPPS114 (salt-tolerant), OM6967 (moderately tolerance), VD20 (salt-sensitive). Increasing salt concentration leads to a reduction in shoot/root length but different levels among the cultivars. In contrast, reactive oxygen species (ROS) accumulation and lipid peroxidation increased progressively with increasing salt concentration and time course treatment. However, at 250 ?M of NaCl, these parameters were more adversely affected in VD20 than AGPPS114 and OM6967. Using ICP showed that Na+ accumulation in rice root increased gradually with increasing NaCl concentrations in all cultivars under salt treatment but was low in salt-sensitive cultivar VD20 compared to other cultivars. Antioxidant enzyme activity analysis indicated catalase (CAT) and superoxide dismutase (SOD) were induced during salt treatment in all cultivars. The results also showed greater proline and glycine betaine accumulation in the AGPPS114 than OM6976 and VD20. qPCR indicated a significant difference in transcript levels of the Na+-transporter gene OsSOS1, OsNHX1 and OsHKT1s in AGPPS114 and OM6967 cultivars compared to VD20 cultivar. In summary, the active regulation of genes related to Na+ transport at the transcription level and with high glycine betaine and proline accumulation levels may be involved in salt tolerance mechanisms and thus might be useful for selecting tolerant plants.
Downloads
References
Jesus JM, Danko AS, Fiúza A, Borges MT. Phytoremediation of salt-affected soils: a review of processes, applicability and the impact of climate change. Environ Sci Pollut Res. 2015;22(9):6511-25. http://doi.org/10.1007/s11356-015-4205-4
Kumar K, Kumar M, Kim SR, Ryu H, Cho YG. Insights into genomics of salt stress response in rice. Rice. 2013;6(1):27. http://doi.org/10.1186/1939-8433-6-27
Baca AC, Nguyen DH, Srivastava JP, Hanumappa M, Wilderspin IF, Chinh NC et al. Toward integrated disaster risk management in Vietnam: recommendations based on the drought and saltwater intrusion crisis and the case for investing in longer-term resilience - overview. Washington, D.C.: World Bank Group; 2017.
GriSP GRSP. GRiSP in Motion, Annual Report 2012. Los Banos, Philippines: International Rice Research Institure; 2013.
Hussain A, Shahzad A, Tabassum S, Hafeez H, Khattak JZK. Salt stress tolerance of transgenic rice (Oryza sativa L.) expressing AtDREB1A gene under inducible or constitutive promoters. Biologia. 2018;73(1):31-41. http://doi.org/10.2478/s11756-018-0010-0
Yeo AR, Flowers TJ. Accumulation and localisation of sodium ions within the shoots of rice (Oryza sativa) varieties differing in salinity resistance. Physiol Plant. 1982;56(3):343-48. http://doi.org/10.1111/j.1399-3054.1982.tb00350.x
Moradi F, Ismail A, Egdane J, Gregorio G. Salinity tolerance of rice during reproductive development and association with tolerance at the seedling stage. Indian Journal of Plant Physiology. 2003;8:105-16.
Walia H, Wilson C, Condamine P, Liu X, Ismail AM, Zeng L et al. Comparative transcriptional profiling of two contrasting rice genotypes under salinity stress during the vegetative growth stage. Plant Physiol. 2005;139(2):822-35. http://doi.org/10.1104/pp.105.065961
Bonilla P, Dvorak J, Mackell D, Deal K, Gregorio G. RFLP and SSLP mapping of salinity tolerance genes in chromosome 1 of rice (Oryza sativa L.) using recombinant inbred lines. Philippine Agricultural Scientist (Philippines). 2002.
Noctor G, Foyer CH. Ascorbate and glutathione: keeping active oxygen under control. Annu Rev Plant Biol. 1998;49(1):249-79. https://doi.org/10.1146/annurev.arplant.49.1.249
Dat J, Vandenabeele S, Vranova E, Van Montagu M, Inzé D, Van Breusegem F. Dual action of the active oxygen species during plant stress responses. Cellular and Molecular Life Sciences, CMLS. 2000;57(5):779-95. https://doi.org/10.1007/s000180050041
Xie X, He Z, Chen N, Tang Z, Wang Q, Cai Y. The roles of environmental factors in regulation of oxidative stress in plant. Biomed Res Int. 2019;2019:9732325-. http://doi.org/10.1155/2019/9732325
Kawasaki S, Borchert C, Deyholos M, Wang H, Brazille S, Kawai K et al. Gene expression profiles during the initial phase of salt stress in rice. Plant Cell. 2001;13(4):889-905. http://doi.org/10.2307/3871347
Barkla BJ, Vera-Estrella R, Pantoja O. Towards the production of salt-tolerant crops. In: Shahidi F, Kolodziejczyk P, Whitaker JR, Munguia AL, Fuller G, editors. Chemicals via Higher Plant Bioengineering. Boston, MA: Springer US; 1999. p. 77-89. https://doi.org/10.1007/978-1-4615-4729-7_7
Sakamoto A, Murata N. Genetic engineering of glycinebetaine synthesis in plants: current status and implications for enhancement of stress tolerance. J Exp Bot. 2000;51(342):81-88. http://doi.org/10.1093/jexbot/51.342.81
Hasegawa PM, Bressan RA, Zhu J-K, Bohnert HJ. Plan cellular and molecular responses to high salinity. Annu Rev Plant Physiol Plant Mol Biol. 2000;51(1):463-99. http://doi.org/10.1146/annurev.arplant.51.1.463
Yamaguchi T, Blumwald E. Developing salt-tolerant crop plants: challenges and opportunities. Trends in Plant Science. 2005;10(12):615-20. http://doi.org/https://doi.org/10.1016/j.tplants.2005.10.002
Nuccio ML, Paul M, Bate NJ, Cohn J, Cutler SR. Where are the drought tolerant crops? An assessment of more than two decades of plant biotechnology effort in crop improvement. Plant Science. 2018;273:110-19. http://doi.org/https://doi.org/10.1016/j.plantsci.2018.01.020
Jaspers P, Kangasjärvi J. Reactive oxygen species in abiotic stress signaling. Physiol Plant. 2010;138(4):405-13. http://doi.org/10.1111/j.1399-3054.2009.01321.x
Hirayama T, Shinozaki K. Research on plant abiotic stress responses in the post-genome era: past, present and future. Plant J. 2010;61(6):1041-52. http://doi.org/10.1111/j.1365-313X.2010.04124.x
Lindemose S, Shea C, Jensen MK, Skriver K. Structure, function and networks of transcription factors involved in abiotic stress responses. International Journal of Molecular Sciences. 2013;14(3):5842-78. https://doi.org/10.3390/ijms14035842
Reddy INBL, Kim BK, Yoon IS, Kim KH, Kwon TR. Salt tolerance in rice: Focus on mechanisms and approaches. Rice Science. 2017;24(3):123-44. http://doi.org/https://doi.org/10.1016/j.rsci.2016.09.004
El Mahi H, Pérez-Hormaeche J, De Luca A, Villalta I, Espartero J, Gámez-Arjona F et al. A critical role of sodium flux via the plasma membrane Na+/H+ exchanger SOS1 in the salt tolerance of rice. Plant Physiol. 2019;180(2):1046-65. http://doi.org/10.1104/pp.19.00324
Ren ZH, Gao JP, Li LG, Cai XL, Huang W, Chao DY et al. A rice quantitative trait locus for salt tolerance encodes a sodium transporter. Nature Genetics. 2005;37(10):1141-46. http://doi.org/10.1038/ng1643
Yao X, Horie T, Xue S, Leung H-Y, Katsuhara M, Brodsky DE et al. Differential sodium and potassium transport selectivities of the rice OsHKT2; 1 and OsHKT2; 2 transporters in plant cells. Plant Physiol. 2010;152(1):341-55. https://doi.org/10.1104/pp.109.145722
Hussain S, Cao X, Zhong C, Zhu L, Khaskheli MA, Fiaz S et al. Sodium chloride stress during early growth stages altered physiological and growth characteristics of rice. Chilean Journal of Agricultural Research. 2018;78:183-97. https://doi.org/10.4067/S0718-58392018000200183
Tahjib-Ul-Arif M, Sayed MA, Islam MM, Siddiqui MN, Begum SN, Hossain MA. Screening of rice landraces (Oryza sativa L.) for seedling stage salinity tolerance using morpho-physiological and molecular markers. Acta Physiol Plant. 2018;40(4):70. http://doi.org/10.1007/s11738-018-2645-4
Khan MH, Panda SK. Alterations in root lipid peroxidation and antioxidative responses in two rice cultivars under NaCl-salinity stress. Acta Physiol Plant. 2007;30(1):81. http://doi.org/10.1007/s11738-007-0093-7
International Rice Research Institute. Standard evaluation system for rice. Manila, Philippine: Genetic Resources Centre, IRRI; 1996.
Ministry-of-Agricultural-and-Rural-Development. Rice breeding and crop seasons in Mekong River Delta, Vietnam. Ha Noi, Vietnam: Nong nghiep Publisher; 2006. 30 p.
Huynh-Van-Thon, Duong-Van-Chin. The results in high technology application in rice crop production of Loc Troi Group. In: Commission CE, Vietnam AE, Editors. High quality and sustainable agricultural development in Vietnam; Ha Noi 2017. p. 370.
Oh DH, Leidi E, Zhang Q, Hwang SM, Li Y, Quintero FJ, et al. Loss of Halophytism by Interference with SOS1 Expression. Plant Physiol. 2009;151(1):210-22. http://doi.org/10.1104/pp.109.137802
Thordal-Christensen H, Yangdou Wei Z, Collinge D. Subcellular localization of H2O2 in plants. H2O2 accumulation in papillae and hypersensitive response during the barley-powdery mildew interaction. Plant J. 1997;11(6):1187 - 94. https://doi.org/10.1046/j.1365-313X.1997.11061187.x
Pompella A, Maellaro E, Casini AF, Comporti M. Histochemical detection of lipid-peroxidation in the liver of bromobenzene-poisoned mice. The American Journal of Pathology. 1987;129(2):295-301.
Yamamoto Y, Kobayashi Y, Matsumoto H. Lipid peroxidation is an early symptom triggered by aluminum, but not the primary cause of elongation inhibition in pea roots. Plant Physiol. 2001;125(1):199-208. http://doi.org/10.1104/pp.125.1.199
Bates LS, Waldren RP, Teare ID. Rapid determination of free proline for water-stress studies. Plant Soil. 1973;39(1):205-57. http://doi.org/10.1007/BF00018060
Grieve C, Grattan S. Rapid assay for determination of water soluble quaternary ammonium compounds. Plant Soil. 1983;70(2):303-07. https://doi.org/10.1007/BF02374789
Yeh CM, Chien PS, Huang HJ. Distinct signalling pathways for induction of MAP kinase activities by cadmium and copper in rice roots. J Exp Bot. 2007;58(3):659-71. http://doi.org/10.1093/jxb/erl240
Beauchamp C, Fridovich I. Superoxide dismutase: Improved assays and an assay applicable to acrylamide gels. Anal Biochem. 1971;44(1):276-87. http://doi.org/https://doi.org/10.1016/0003-2697(71)90370-8
Beers R, Sizer J. Colorimetric method for estimation of catalase. J Biol Chem. 1952;195:133-39. https://doi.org/10.1016/S0021-9258(19)50881-X
Wang Y-S, Yang Z-M. Nitric oxide reduces aluminum toxicity by preventing oxidative stress in the roots of Cassia tora L. Plant and Cell Physiology. 2005;46(12):1915-23. http://doi.org/10.1093/pcp/pci202
Livak KJ, Schmittgen TD. Analysis of relative gene expression data using real-time quantitative PCR and the 2???CT method. Methods. 2001;25(4):402-48. http://doi.org/http://dx.doi.org/10.1006/meth.2001.1262
Shereen A, Mumtaz S, Raza S, Khan M, Solangi S. Salinity effects on seedling growth and yield components of different inbred rice lines. Pakistan Journal of Botany. 2005;37(1):131-39.
Darwish E, Testerink C, Khalil M, El-Shihy O, Munnik T. Phospholipid signaling responses in salt-stressed rice leaves. Plant and Cell Physiology. 2009;50(5):986-97. https://doi.org/10.1093/pcp/pcp051
Oyiga BC, Sharma RC, Baum M, Ogbonnaya FC, Léon J, Ballvora A. Allelic variations and differential expressions detected at quantitative trait loci for salt stress tolerance in wheat. Plant, Cell and Environment. 2018;41(5):919-35. http://doi.org/10.1111/pce.12898
Danai-Tambhale S, Kumar V, Shriram V. Differential response of two scented indica rice (Oryza sativa) cultivars under salt stress. Journal of Stress Physiology & Biochemistry. 2011;7:387-97.
Akbar M, Ponnamperuma F. Saline soils of South and Southeast Asia as potential rice lands. Rice research strategies for the future IRRI. 1982:265-81.
Anbumalarmathi J, Mehta P. Effect of salt stress on germination of indica rice varieties. European Journal of Biological Science. 2013;6(1):1-6.
Dadkhah A, Stewart W, Griffith H. Effects of salinity on yield and root quality of sugar beet (Beta vulgaris L.). J Exp Bot. 2001;52:31.
Momayezia MR, Editor Effect of chloride and sulfate salinity on nutrient uptake in Iranian rice (Oryza sativa L.). 19th World Congress of Soil Science; 2010.
Petrov V, Hille J, Mueller-Roeber B, Gechev TS. ROS-mediated abiotic stress-induced programmed cell death in plants. Frontiers in Plant Science. 2015;6:69-. http://doi.org/10.3389/fpls.2015.00069
Mittova V, M. T, M V, M. G. Up-regulation of the leaf mitochondrial and peroxisomal antioxidative systems in response to salt-induced oxidative stress in the wild salt-tolerant tomato species Lycopersicon pennellii. Plant, Cell and Environment. 2003;6:845-56. https://doi.org/10.1046/j.1365-3040.2003.01016.x
Demiral T, Türkan ?. Comparative lipid peroxidation, antioxidant defense systems and proline content in roots of two rice cultivars differing in salt tolerance. Environmental and Experimental Botany. 2005;53(3):247-57. http://doi.org/https://doi.org/10.1016/j.envexpbot.2004.03.017
Hoque MA, Banu MNA, Nakamura Y, Shimoishi Y, Murata Y. Proline and glycinebetaine enhance antioxidant defense and methylglyoxal detoxification systems and reduce NaCl-induced damage in cultured tobacco cells. J Plant Physiol. 2008;165(8):813-24. http://doi.org/https://doi.org/10.1016/j.jplph.2007.07.013
Banu MNA, Hoque MA, Watanabe-Sugimoto M, Matsuoka K, Nakamura Y, Shimoishi Y et al. Proline and glycinebetaine induce antioxidant defense gene expression and suppress cell death in cultured tobacco cells under salt stress. J Plant Physiol. 2009;166(2):146-56. http://doi.org/https://doi.org/10.1016/j.jplph.2008.03.002
El-Shabrawi H, Kumar B, Kaul T, Reddy MK, Singla-Pareek SL, Sopory SK. Redox homeostasis, antioxidant defense and methylglyoxal detoxification as markers for salt tolerance in Pokkali rice. Protoplasma. 2010;245(1-4):85-96. https://doi.org/10.1007/s00709-010-0144-6
Hasanuzzaman M, Alam M, Rahman A, Hasanuzzaman M, Nahar K, Fujita M. Exogenous proline and glycine betaine mediated upregulation of antioxidant defense and glyoxalase systems provides better protection against salt-induced oxidative stress in two rice (Oryza sativa L.) varieties. Biomed Res Int. 2014;2014:1-17. https://doi.org/10.1155/2014/757219
Kishor PBK, Sreenivasulu N. Is proline accumulation per se correlated with stress tolerance or is proline homeostasis a more critical issue? Plant, Cell and Environment. 2014;37(2):300-11. http://doi.org/10.1111/pce.12157
Kishitani S, Takanami T, Suzuki M, Oikawa M, Yokoi S, Ishitani M et al. Compatibility of glycinebetaine in rice plants: evaluation using transgenic rice plants with a gene for peroxisomal betaine aldehyde dehydrogenase from barley. Plant, Cell and Environment. 2000;23(1):107-14. https://doi.org/10.1046/j.1365-3040.2000.00527.x
Wutipraditkul N, Wongwean P, Buaboocha T. Alleviation of salt-induced oxidative stress in rice seedlings by proline and/or glycinebetaine. Biologia Plantarum. 2015;59(3):547-53. http://doi.org/10.1007/s10535-015-0523-0
Malekzadeh P. Influence of exogenous application of glycinebetaine on antioxidative system and growth of salt-stressed soybean seedlings (Glycine max L.). Physiology and Molecular Biology of Plants. 2015;21(2):225-32. http://doi.org/10.1007/s12298-015-0292-4
Martínez-Atienza J, Jiang X, Garciadeblas B, Mendoza I, Zhu JK, Pardo JM et al. Conservation of the salt overly sensitive pathway in rice. Plant Physiol. 2007;143(2):1001-12. http://doi.org/10.1104/pp.106.092635
Ji H, Pardo JM, Batelli G, Van Oosten MJ, Bressan RA, Li X. The Salt Overly Sensitive (SOS) pathway: established and emerging roles. Mol Plant. 2013;6(2):275-86. http://doi.org/10.1093/mp/sst017
Jiang X, Leidi EO, Pardo JM. How do vacuolar NHX exchangers function in plant salt tolerance? Plant Signal Behav. 2010;5(7):792-95. http://doi.org/10.4161/psb.5.7.11767
Fukuda A, Nakamura A, Hara N, Toki S, Tanaka Y. Molecular and functional analyses of rice NHX-type Na+/H+ antiporter genes. Planta. 2011;233(1):175-88. http://doi.org/10.1007/s00425-010-1289-4
Theerawitaya C, Tisarum R, Samphumphuang T, Takabe T, Cha-Um S. Expression levels of the Na(+)/K(+) transporter OsHKT2;1 and vacuolar Na(+)/H(+) exchanger OsNHX1, Na enrichment, maintaining the photosynthetic abilities and growth performances of indica rice seedlings under salt stress. Physiology and Molecular Biology of Plants. 2020;26(3):513-23. http://doi.org/10.1007/s12298-020-00769-3
Yokoi S, Quintero FJ, Cubero B, Ruiz MT, Bressan RA, Hasegawa PM, et al. Differential expression and function of Arabidopsis thaliana NHX Na+/H+ antiporters in the salt stress response. Plant Journal. 2002;30(5):529-39. http://doi.org/10.1046/j.1365-313x.2002.01309.x
Ye CY, Zhang HC, Chen JH, Xia XL, Yin WL. Molecular characterization of putative vacuolar NHX-type Na+/H+ exchanger genes from the salt-resistant tree Populus euphratica. Physiol Plant. 2009;137(2):166-74. http://doi.org/10.1111/j.1399-3054.2009.01269.x
Fukuda A, Nakamura A, Tagiri A, Tanaka H, Miyao A, Hirochika H et al. Function, intracellular localization and the importance in salt tolerance of a vacuolar Na(+)/H(+) antiporter from rice. Plant Cell Physiol. 2004;45(2):146-59. http://doi.org/10.1093/pcp/pch014
Shi H, Ishitani M, Kim C, Zhu J-K. The Arabidopsis thaliana salt tolerance gene SOS1 encodes a putative Na+/H+ antiporter. Proc Natl Acad Sci USA. 2000;97(12):6896-901. http://doi.org/10.1073/pnas.120170197
Rus A, Lee BH, Muñoz-Mayor A, Sharkhuu A, Miura K, Zhu JK, et al. AtHKT1 facilitates Na+ homeostasis and K+ nutrition in planta. Plant Physiol. 2004;136(1):2500-11. http://doi.org/10.1104/pp.104.042234
Roy SJ, Negrão S, Tester M. Salt resistant crop plants. Current Opinion in Biotechnology. 2014;26:115-24. http://doi.org/https://doi.org/10.1016/j.copbio.2013.12.004
Mishra P, Mishra V, Singh NK, Rai V. Gene expression dynamics of HKT family genes in salt-tolerant and salt-sensitive indica rice cultivars. Indian J Genet. 2017;77(3):364-70. https://doi.org/10.5958/0975-6906.2017.00049.9
Kader MA, Seidel T, Golldack D, Lindberg S. Expressions of OsHKT1, OsHKT2, and OsVHA are differentially regulated under NaCl stress in salt-sensitive and salt-tolerant rice (Oryza sativa L.) cultivars. J Exp Bot. 2006;57(15):4257-68. https://doi.org/10.1093/jxb/erl199
Garciadeblás B, Senn ME, Bañuelos MA, Rodríguez-Navarro A. Sodium transport and HKT transporters: the rice model. Plant J. 2003;34(6):788-801. http://doi.org/10.1046/j.1365-313X.2003.01764.x
Maathuis FJ, Ahmad I, Patishtan J. Regulation of Na+ fluxes in plants. Frontiers in plant science. 2014;5:467. https://doi.org/10.3389/fpls.2014.00467
Horie T, Yoshida K, Nakayama H, Yamada K, Oiki S, Shinmyo A. Two types of HKT transporters with different properties of Na+ and K+ transport in Oryza sativa. Plant J. 2001;27(2):129-38. http://doi.org/10.1046/j.1365-313x.2001.01077.x
Cotsaftis O, Plett D, Shirley N, Tester M, Hrmova M. A two-staged model of Na+ exclusion in rice explained by 3D modeling of HKT transporters and alternative splicing. PloS one. 2012;7(7):e39865. http://doi.org/10.1371/journal.pone.0039865
Shohan MUS, Sinha S, Nabila FH, Dastidar SG, Seraj ZI. HKT1;5 Transporter gene expression and association of samino acid substitutions with salt tolerance across rice genotypes. Frontiers in Plant Science. 2019;10(1420). http://doi.org/10.3389/fpls.2019.01420
Kader MA, Lindberg S. Uptake of sodium in protoplasts of salt-sensitive and salt-tolerant cultivars of rice, Oryza sativa L. determined by the fluorescent dye SBFI. J Exp Bot. 2005;56(422):3149-58. http://doi.org/10.1093/jxb/eri312
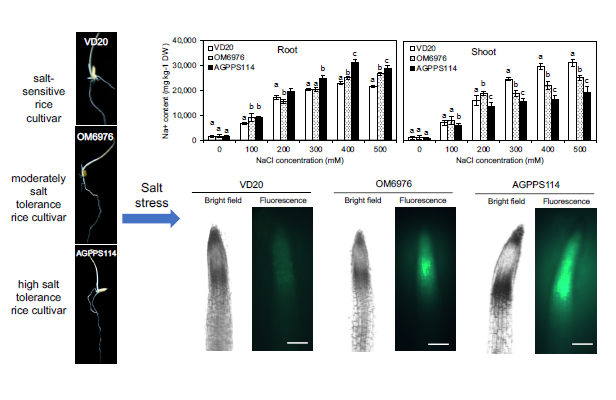
Downloads
Published
How to Cite
Issue
Section
License
Copyright (c) 2022 Nam Trinh Ngoc, Phuong Nguyen Tri, Thia Le Hong, Cuong Duong Quoc

This work is licensed under a Creative Commons Attribution 4.0 International License.
Copyright and Licence details of published articles
Authors who publish with this journal agree to the following terms:
- Authors retain copyright and grant the journal right of first publication with the work simultaneously licensed under a Creative Commons Attribution License that allows others to share the work with an acknowledgement of the work's authorship and initial publication in this journal.
- Authors are able to enter into separate, additional contractual arrangements for the non-exclusive distribution of the journal's published version of the work (e.g., post it to an institutional repository or publish it in a book), with an acknowledgement of its initial publication in this journal.
Open Access Policy
Plant Science Today is an open access journal. There is no registration required to read any article. All published articles are distributed under the terms of the Creative Commons Attribution License (CC Attribution 4.0), which permits unrestricted use, distribution, and reproduction in any medium, provided the original author and source are credited (https://creativecommons.org/licenses/by/4.0/). Authors are permitted and encouraged to post their work online (e.g., in institutional repositories or on their website) prior to and during the submission process, as it can lead to productive exchanges, as well as earlier and greater citation of published work (See The Effect of Open Access).