CO2 sequestration: microalgae genome analysis and its application of effective green source technology
DOI:
https://doi.org/10.14719/pst.1365Keywords:
Carbon assimilation, Carbonic anhydrase, Genomic resources, Green-Gene technology, Microalgal genomes, RuBisCOAbstract
Microalgae genome technology for CO2 sequestration is an appropriate vehicle for articulating the importance of the current need and solution for reduction of CO2 at the atmospheric level. In comparison with C4 plants, microalgae have greater capability to fix atmospheric CO2.The rate of CO2 fixation differs in different strains of microalgae. The photosynthetic enzyme RuBisCO is widely responsible for photosynthetic carbon assimilation in all plants including phototrophic algae.The gene rbcL encodes this enzyme. The catalytic activity of carbonic anhydrase achieves the CO2 generation in the RuBisCO. CAH3 gene is essential for generating CO2 concentration for RuBisCO by dehydration of accumulated inorganic carbon. There are also few other microalgae genes which involves for carbon assimilation. Genomic resource databases and several other nucleotide databases are being used for sequencing the microalgal genomes. Even though, recent advances in genomic studies are providing thrust to enhance the research on microalgal species, they are expensive and resources available for microalgal genomic studies are limited. This review article attempts first as a combined revise on microalgae CO2 sequestration in the field of basic science, applied aspects, and the role of specific gene(s) in the algal system is well defined which could be a supportive involvement of carbon dioxide reduction as “Green-Gene Technology”. This Green biotechnology could be used for Global warming reduction as well as creating wealth from the waste through valuable by-products from the selected microalgae strains in future.
Downloads
References
Saklani N, Khurana A. Global Warming: Effect on Living Organisms, Causes and its Solutions. Int J Eng Manag Res. 2019;09(05):24–6.
Sivaramanan S. Global warming and Climate change. Int J Glob Sci Res. 2019;6(2).
Umair Shahzad R. Global Warming – Causes, Effects and Solution’S Trials. JES J Eng Sci. 2012;40(4):1233–54.
Liu Y, Zhou ZUW, Hong-Cai. Recent advances in carbon dioxide capture with metal-organic frameworks. Greenh Gases Sci Technol. 2012;2(5):352–68.
Habib MA, Badr HM, Ahmed SF, Ben?Mansour R, Mezghani K, Imashuku S, et al. A review of recent developments in carbon capture utilizing oxy?fuel combustion in conventional and ion transport membrane systems. Int J energy Res [Internet]. 2011;35(9):741–64. Available from: https://onlinelibrary.wiley.com/doi/epdf/10.1002/er.1798
Meis NNAH, Bitter JH, Jong KP de. Support and Size Effects of Activated Hydrotalcites for Precombustion CO2 Capture. Ind Eng Chem Res [Internet]. 2010;49:1229-1235. Available from: https://pubs.acs.org/doi/10.1021/ie901114d
Rubin ES, Mantripragada H, Marks A, Versteeg P, Kitchin J. The outlook for improved carbon capture technology. Prog Energy Combust Sci [Internet]. 2012;38(5):630–71. Available from: http://dx.doi.org/10.1016/j.pecs.2012.03.003
Thiruvenkatachari R, Su S, An H, Yu X. Post combustion CO2 capture by carbon fibre monolithic adsorbents. Prog Energy Combust Sci [Internet]. 2009;35(5):438–55. Available from: https://www.cheric.org/research/tech/periodicals/view.php?seq=1334808
Liang Y, Harrison DP, Gupta RP, Green DA, McMichael WJ. Carbon Dioxide Capture Using Dry Sodium-Based Sorbents. Energy Fuels [Internet]. 2004;18(2):569–575. Available from: https://pubs.acs.org/doi/10.1021/ef030158f
Abanades JC, Alonso M, Rodríguez N. Biomass combustion with in situ CO2 capture with CaO. I. process description and economics. Ind Eng Chem Res. 2011;50(11):6972–81.
Qi G, Wang Y, Estevez L, Duan X, Anako N, Park A-HA, et al. High efficiency nanocomposite sorbents for CO2 capture based on amine-functionalized mesoporous capsules. Energy Environ Sci, [Internet]. 2011;4:444–52. Available from: https://pubs.rsc.org/en/content/articlelanding/2011/ee/c0ee00213e#!divAbstract
Wang Y, Duanmu D, Spalding MH. Carbon dioxide concentrating mechanism in Chlamydomonas reinhardtii: Inorganic carbon transport and CO2 recapture. Photosynth Res. 2011;109(1–3):115–22.
Jones CW. CO2 capture from dilute gases as a component of modern global carbon management. Annu Rev Chem Biomol Eng. 2011;2:31–52.
Kenarsari SD, Yang D, Jiang G, Zhang S, Wang J, Russell AG, et al. Review of recent advances in carbon dioxide separation and capture. RSC Adv. 2013;3(45):22739–73.
Kobayashi K, Uno Y. Influence of alkali on carbonation of concrete. Part 1 – Preliminary tests with mortar specimens. Cem Concr Res [Internet]. 1989;19(5):821–826. Available from: https://www.sciencedirect.com/science/article/abs/pii/0008884689900537
Jang JG, Kim GM, Kim HJ, Lee HK. Review on recent advances in CO2 utilization and sequestration technologies in cement-based materials. Constr Build Mater [Internet]. 2016;127(30):762–73. Available from: https://www.sciencedirect.com/science/article/abs/pii/S0950061816316300
Jang. J, H K, K K, K L. Resistance of coal bottom ash mortar against the coupled deterioration of carbonation and chloride penetration. Mater Des [Internet]. 2016;93:160–7. Available from: http://dx.doi.org/10.1016/j.matdes.2015.12.074
Fernández Bertos M, Simons SJR, Hills CD, Carey PJ. A review of accelerated carbonation technology in the treatment of cement-based materials and sequestration of CO2. J Hazard Mater. 2004;112(3):193–205.
Lange LC, Hills CD, Poole AB. The effect of accelerated carbonation on the properties of cement-solidified waste forms. Waste Manag [Internet]. 1996;16(8):757–63. Available from: https://www.sciencedirect.com/science/article/abs/pii/S0956053X97000226
Freund P, Ormerod WG. Progress towards storage of carbon dioxide. Energy Convers Manag. 1997;38:198–205.
Lal R. Carbon sequestration. Philos Trans R Soc B Biol Sci. 2008;363(1492):815–30.
Kerr RA. Bush Backs Spending for a “Global Problem.” Science. 2001 Jun;1982–3. Available from: http://ubc.summon.serialssolutions.com/2.0.0/link/0/eLvHCXMwXZ1NCgIxDIWLeAJBcekFRtp0-rcWBw8wF0ibZOHC-y99Ay7UMyS8770QEuciXf30pwnV5kaGikuXYrAQhami4qUTy6z-Z1XnS-CXg9vp6-jW5b7eHtPnP8D0TNtUr9cqlMwzkQ4V3m7LGRwIoNQEoJWkIfFAUw_kIICIOEdTBJJRsrd4cntEbD27SwO2KlAH78S
O’Connor WK, Dahlin DC, NilsenD.N., Rush GE, Walters RP, Turner PC. Carbon Dioxide Sequestration by Direct Mineral Carbonation: Results from Recent Studies and Current Status. In: 1st Annual DOE Carbon Sequestration Conference, Washington, DC. Washington, D.C; 2001. p. 11.
Tsang CF, Benson SM, Kogelski B, Smith RE. Scientific considerations related to regulation development for CO2 sequestration in brine formations. Environ Geol. 2002;42:275–281.
Klara SM, Srivastava RD, McIlvried HG. Integrated collaborative technology development program for CO 2 sequestration in geologic formations - United States Department of Energy R&D. Energy Convers Manag. 2003;44(17):2699–712.
Baines SJ, Worden RH. Geological storage of carbon dioxide. Geol Soc Spec Publ. 2004;233(June):1–6.
Kintisch E. Report Backs More Projects to Sequester CO 2 From Coal. Science [Internet]. 2007;315(5818):1481. Available from: https://science.sciencemag.org/content/315/5818/1481.1/tab-e-letters
Schrag DP. Preparing to capture carbon. Science (80- ) [Internet]. 2007;9(315(5813)):812–3. Available from: https://pubmed.ncbi.nlm.nih.gov/17289991/
Klusman RW. Evaluation of leakage potential CO2 EOR/sequestration project. Energy Convers Manag. 2003;33:1921–1940.
Lackner KS. A guide to CO2 sequestration. Science (80- ). 2003;300(5626):1677–8.
Park A-HA, Fan L-S. CO2 mineral sequestration: Physically activated dissolution of serpentine and pH swing process. Chem Eng Sci. 2004;59(22–23):5241–7.
Rivkin RB, Legendre L. Biogenic carbon cycling in the upper ocean: Effects of microbial respiration. Science (80- ). 2001;291(5512):2398–400.
Falkowski P, Scholes RJ, Boyle E, Canadell J, Canfield D, Elser J, et al. The global carbon cycle: A test of our knowledge of earth as a system. Science (80- ). 2000;290(5490):291–6.
Raven JA, Falkowski PG. Oceanic sinks for atmospheric CO2. Plant, Cell Environ. 1999;22(6):741–55.
Falkowski PG. Letters to nature. Nature. 1997;387:243–4.
Martin JH, Fitzwater SE. Iron deficiency limits phytoplankton growth in the north-east Pacific subarctic. Nature [Internet]. 1988;331(6154):341–3. Available from: https://app.dimensions.ai/details/publication/pub.1002094778
Boyd P, Law C, Wong C, Al. E. The decline and fate of an iron-induced subarctic phytoplankton bloom. Nature [Internet]. 2004;428:549–553. Available from: https://www.nature.com/articles/nature02437#citeas
Lal R, Follett RF, Kimble JM. Achieving Soil Carbon Sequestration in the United States: A Challenge to the Policy Makers. Soil Sci [Internet]. 2003;168(12):827–45. Available from: https://journals.lww.com/soilsci/Abstract/2003/12000/ACHIEVING_SOIL_CARBON_SEQUESTRATION_IN_THE_UNITED.1.aspx
Figueroaa DJ, TimothyFouta, SeanPlasynskia, HowardMcIlvriedb, Rameshwar. Advances in CO2 capture technology—The U.S. Department of Energy’s Carbon Sequestration Program. Greenh Gas Control [Internet]. 2008;2(1):9–20. Available from: https://www.sciencedirect.com/science/article/abs/pii/S1750583607000941
Pires JCM, Martins FG, Alvim-Ferraz MCM, Simões M. Recent developments on carbon capture and storage: An overview. Chem Eng Res Des [Internet]. 2011;89(9):1446–60. Available from: http://dx.doi.org/10.1016/j.cherd.2011.01.028
Pires JCM, Alvim-Ferraz MCM, Martins FG, Simões M. Carbon dioxide capture from flue gases using microalgae: Engineering aspects and biorefinery concept. Renew Sustain Energy Rev [Internet]. 2012;16(5):3043–53. Available from: http://dx.doi.org/10.1016/j.rser.2012.02.055
Svenssona R, Odenbergera M, Johnssona F, Strömberg L. Transportation systems for CO2––application to carbon capture and storage. Energy Convers Manag [Internet]. 2004;45(15–16):2343–53. Available from: https://www.sciencedirect.com/science/article/abs/pii/S0196890403003662
McCoy ST, Rubin ES. An engineering-economic model of pipeline transport of CO2 with application to carbon capture and storage. Int J Greenh Gas Control. 2008;2(2):219–29.
Lam MK, Lee KT, Mohamed AR. Current status and challenges on microalgae-based carbon capture. Int J Greenh Gas Control [Internet]. 2012;10:456–69. Available from: https://www.sciencedirect.com/science/article/abs/pii/S1750583612001673
De Silva GPD, Ranjith PG, Perera MSA. Geochemical aspects of CO2 sequestration in deep saline aquifers: A review. Fuel [Internet]. 2015;155:128–43. Available from: http://dx.doi.org/10.1016/j.fuel.2015.03.045
Nouha K, John RP, Yan S, Tyagi RD. Carbon Capture and Sequestration: Biological Technologies. In: Surampalli RY, Zhang TC, Tyagi RD, Ravi Naidu BR, Gurjar CS, Ojha P, et al., editors. Carbon Capture and Storage - Physical, Chemical, and Biological Methods [Internet]. Reston, VA 20191-4400: American Society of Civil Engineers; 2015. Available from: https://app.knovel.com/web/toc.v/cid:kpCCSPCBM8/viewerType:toc/
Farrelly DJ, Everard CD, Fagan CC, McDonnell KP. Carbon sequestration and the role of biological carbon mitigation: A review. Renew Sustain Energy Rev [Internet]. 2013;21:712–27. Available from: http://dx.doi.org/10.1016/j.rser.2012.12.038
Cheah WY, Ling TC, Juan JC, Lee DJ, Chang JS, Show PL. Biorefineries of carbon dioxide: From carbon capture and storage (CCS) to bioenergies production. Bioresour Technol [Internet]. 2016;215:346–56. Available from: http://dx.doi.org/10.1016/j.biortech.2016.04.019
Williamson P, Wallace DWR, Law CS, Boyd PW, Collos Y, Croot P, et al. Ocean fertilization for geoengineering: A review of effectiveness, environmental impacts and emerging governance. Process Saf Environ Prot [Internet]. 2012;90(6):475–88. Available from: http://dx.doi.org/10.1016/j.psep.2012.10.007
Yadav G, Sen R. Microalgal green refinery concept for biosequestration of carbon-dioxide vis-à-vis wastewater remediation and bioenergy production: Recent technological advances in climate research. J CO2 Util [Internet]. 2017;17:188–206. Available from: http://dx.doi.org/10.1016/j.jcou.2016.12.006
Zhou W, Wang J, Chen P, Ji C, Kang Q, Lu B, et al. Bio-mitigation of carbon dioxide using microalgal systems: Advances and perspectives. Renew Sustain Energy Rev [Internet]. 2017;76(March):1163–75. Available from: http://dx.doi.org/10.1016/j.rser.2017.03.065
Singh J, Dhar DW. Overview of carbon capture technology: Microalgal biorefinery concept and state-of-the-art. Front Mar Sci. 2019;6(FEB):1–9.
Slade R, Bauen A. Micro-algae cultivation for biofuels: Cost, energy balance, environmental impacts and future prospects. Biomass and Bioenergy [Internet]. 2013;53(0):29–38. Available from: http://dx.doi.org/10.1016/j.biombioe.2012.12.019
Valdovinos-García EM, Barajas-Fernández J, de los Ángeles Olán-Acosta M, Petriz-Prieto MA, Guzmán-López A, Bravo-Sánchez MG. Techno-Economic Study of CO2 Capture of a Thermoelectric Plant Using Microalgae (Chlorella vulgaris) for Production of Feedstock for Bioenergy. Energies. 2020;13(2):1–19.
Bhola V, Swalaha F, Ranjith Kumar R, Singh M, Bux F. Overview of the potential of microalgae for CO2 sequestration. Int J Environ Sci Technol. 2014;11(7).
Gaikwad RW, Gudadhe MD, Bhagat SL. Carbon Dioxide Capture , Tolerance and Sequestration Using Microalgae- A Review. Int J Pharm Chem Biol Sci [Internet]. 2016;6(3):345–9. Available from: http://www.ijpcbs.com/files/13-07-2016/13.pdf
Fernández I, Acién FG, Fernández JM, Guzmán JL, Magán JJ, Berenguel M. Bioresource Technology Dynamic model of microalgal production in tubular photobioreactors. 2012;126:172–81.
Costache TA, Gabriel Acien Fernandez F, Morales MM, Fernández-Sevilla JM, Stamatin I, Molina E. Comprehensive model of microalgae photosynthesis rate as a function of culture conditions in photobioreactors. Appl Microbiol Biotechnol. 2013;97(17):7627–37.
Seo SH, Ha JS, Yoo C, Srivastava A, Ahn CY, Cho DH, et al. Light intensity as major factor to maximize biomass and lipid productivity of Ettlia sp. in CO2-controlled photoautotrophic chemostat [Internet]. Vol. 244, Bioresource Technology. Elsevier Ltd; 2017. 621–628 p. Available from: http://dx.doi.org/10.1016/j.biortech.2017.08.020
Vonshak A, Giuseppe T. Environmental Stress Physiology. In: Richmond A, editor. Handbook of Microalgal Culture: Biotechnology and Applied Phycology. Iowa 50014-8300, USA: Blackwell Publishing Ltd; 2004. p. 57–82.
Falkowski P., Chen. Y.B. Photoacclimation of Light Harvesting Systems in Eukaryotic Algae. In: B.R. G, W.W. P, editors. Light-Harvesting Antennas in Photosynthesis Advances in Photosynthesis and Respiration [Internet]. Springer, Dordrecht; 2003. p. 423–47. Available from: https://link.springer.com/chapter/10.1007/978-94-017-2087-8_15#citeas
Nikolaou A, Hartmann P, Sciandra A, Chachuat B, Bernard O. Dynamic coupling of photoacclimation and photoinhibition in a model of microalgae growth. J Theor Biol [Internet]. 2016;390:61–72. Available from: http://dx.doi.org/10.1016/j.jtbi.2015.11.004
Kommareddy A, Anderson G. Study of Light as a parameter in the growth of algae in a Photo-Bioreactor ( PBR ). In: The Society for engineering in agricultural, food, and biological systems [Internet]. Las Vegas, NV; 2003. p. 2–23. Available from: https://www.researchgate.net/publication/280720969_Study_of_Light_as_a_parameter_in_the_growth_of_algae_in_a_Photo-Bioreactor_PBR#:~:text=A photo-bio Reactor is,parameter in a photosynthetic process.&text=Light with wavelengths between 600,the most effici
Luveshan R, Rawat I, Bux F. Light enhancement strategies improve microalgal biomass productivity. Renew Sustain Energy Rev [Internet]. 2017;80:765–73. Available from: https://www.sciencedirect.com/science/article/abs/pii/S1364032117308389
Morales M, Sánchez L, Revah S. The impact of environmental factors on carbon dioxide fixation by microalgae. FEMS Microbiol Lett. 2018;365(3):1–11.
Molina Grima E, Belarbi EH, Acién Fernández FG, Robles Medina A, Chisti Y. Recovery of microalgal biomass and metabolites: Process options and economics. Biotechnol Adv. 2003;20(7–8):491–515.
Béchet Q, Shilton A, Guieysse B. Modeling the effects of light and temperature on algae growth: State of the art and critical assessment for productivity prediction during outdoor cultivation. Biotechnol Adv [Internet]. 2013;31(8):1648–63. Available from: http://dx.doi.org/10.1016/j.biotechadv.2013.08.014
Ördög V, Stirk WA, Bálint P, Aremu AO, Okem A, Lovász C, et al. Effect of temperature and nitrogen concentration on lipid productivity and fatty acid composition in three Chlorella strains. Algal Res [Internet]. 2016;16:141–9. Available from: http://dx.doi.org/10.1016/j.algal.2016.03.001
Kumar A, Ergas S, Yuan X, Sahu A, Zhang Q, Dewulf J, et al. Enhanced CO2 fixation and biofuel production via microalgae: Recent developments and future directions. Trends Biotechnol [Internet]. 2010;28(7):371–80. Available from: http://dx.doi.org/10.1016/j.tibtech.2010.04.004
Ras M, Steyer JP, Bernard O. Temperature effect on microalgae: A crucial factor for outdoor production. Rev Environ Sci Biotechnol. 2013;12(2):153–64.
Zhao B, Su Y. Process effect of microalgal-carbon dioxide fixation and biomass production: A review. Renew Sustain Energy Rev. 2014;31:121–32.
Briassoulis D, Panagakis P, Chionidis M, Tzenos D, Lalos A, Tsinos C, et al. An experimental helical-tubular photobioreactor for continuous production of Nannochloropsis sp. Bioresour Technol [Internet]. 2010;101(17):6768–77. Available from: http://dx.doi.org/10.1016/j.biortech.2010.03.103
Morais MG De, Alberto J, Costa V. Carbon dioxide fixation by Chlorella kessleri , C . vulgaris , Scenedesmus photobioreactors Carbon dioxide fixation by Chlorella kessleri , C . vulgaris , Scenedesmus obliquus and Spirulina sp . cultivated in flasks and vertical tubular photobioreactors. 2007;(October 2014).
Zhang H, Zeng R, Chen D, Liu J. A pivotal role of vacuolar H+-ATPase in regulation of lipid production in Phaeodactylum tricornutum. Sci Rep [Internet]. 2016;6(April):1–17. Available from: http://dx.doi.org/10.1038/srep31319
Moss B. The Influence of Environmental Factors on the Distribution of Freshwater Algae: An Experimental Study: I. Introduction and the Influence of Calcium Concentration. J Ecol [Internet]. 1972;60(3):917–932. Available from: https://www.jstor.org/stable/2258575?seq=1
Azov Y. Effect of pH on Inorganic Carbon Uptake in Algal Cultures. Appl Env Microbiol [Internet]. 1982;43(6):1300–6. Available from: https://pubmed.ncbi.nlm.nih.gov/16346029/
Olaizola M. Commercial development of microalgal biotechnology: From the test tube to the marketplace. Biomol Eng. 2003;20(4–6):459–66.
Tiwari A, Pandey JP, Pathak N. Standardization of pH and Light Intensity for the Biomass Production of Spirulina platensis. J Algal Biomass Utln [Internet]. 2010;2010(2):93–102. Available from: https://www.researchgate.net/publication/299597097
Ying K, Gilmour DJ, Zimmerman WB. Effects of CO2 and pH on growth of the microalga Dunaliella salina. J Microb Biochem Technol. 2014;6(3):167–73.
Duarte-Santos T, Mendoza-Martín JL, Acién Fernández FG, Molina E, Vieira-Costa JA, Heaven S. Optimization of carbon dioxide supply in raceway reactors: Influence of carbon dioxide molar fraction and gas flow rate. Bioresour Technol [Internet]. 2016;212:72–81. Available from: http://dx.doi.org/10.1016/j.biortech.2016.04.023
Bilanovic D, Holland M, Starosvetsky J, Armon R. Co-cultivation of microalgae and nitrifiers for higher biomass production and better carbon capture. Bioresour Technol [Internet]. 2016;220:282–8. Available from: http://dx.doi.org/10.1016/j.biortech.2016.08.083
Solovchenko A, Khozin-Goldberg I. High-CO2 tolerance in microalgae: Possible mechanisms and implications for biotechnology and bioremediation. Biotechnol Lett. 2013;35(11):1745–52.
Cheng L, Zhang L, Chen H, Gao C. Carbon dioxide removal from air by microalgae cultured in a membrane-photobioreactor. Sep Purif Technol. 2006;50(3):324–9.
Yun Y, Lee SB, Park JM, Lee C, Yang J. Carbon Dioxide Fixation by Algal Cultivation Using Wastewater Nutrients. J Chem Technol Biotechnol [Internet]. 1997;69(4):451–5. Available from: https://onlinelibrary.wiley.com/doi/abs/10.1002/%28SICI%291097-4660%28199708%2969%3A4%3C451%3A%3AAID-JCTB733%3E3.0.CO%3B2-M
Chiu SY, Kao CY, Chen CH, Kuan TC, Ong SC, Lin CS. Reduction of CO2 by a high-density culture of Chlorella sp. in a semicontinuous photobioreactor. Bioresour Technol. 2008;99(9):3389–96.
Singh SP, Singh P. Effect of CO2 concentration on algal growth: A review. Renew Sustain Energy Rev. 2014;38:172–9.
Singh UB, Ahluwalia AS. Microalgae: a promising tool for carbon sequestration. Mitig Adapt Strateg Glob Chang [Internet]. 2013;18:73–95. Available from: https://link.springer.com/article/10.1007/s11027-012-9393-3#citeas
Sung KD, Lee JS, Shin CS, Park SC. Isolation of a new highly CO2 tolerant fresh water microalga Chlorella SP. KR-1. Renew Energy. 1999;16(1–4):1019–22.
Sivakumar M, Ranjith Kumar R, Shashirekha V, Seshadri S. Influence of carbon-dioxide on the growth of Spirulina sp. (MCRC-A0003) isolated from Muttukadu backwaters, South India. World J Microbiol Biotechnol. 2014;30(10).
Hanagata N, Takeuchi T, Fukuju Y, Barnes DJ, Karube I. Tolerance of microalgae to high CO2 and high temperature. Phytochemistry. 1992;31(10):3345–8.
Kim W, Park JM, Gim GH, Jeong SH, Kang CM, Kim DJ, et al. Optimization of culture conditions and comparison of biomass productivity of three green algae. Bioprocess Biosyst Eng. 2012;35(1–2):19–27.
Cabello J, Toledo-Cervantes A, Sánchez L, Revah S, Morales M. Effect of the temperature, pH and irradiance on the photosynthetic activity by Scenedesmus obtusiusculus under nitrogen replete and deplete conditions. Bioresour Technol [Internet]. 2015;181:128–35. Available from: http://dx.doi.org/10.1016/j.biortech.2015.01.034
Toledo-Cervantes A, Morales M, Novelo E, Revah S. Carbon dioxide fixation and lipid storage by Scenedesmus obtusiusculus. Bioresour Technol [Internet]. 2013;130:652–8. Available from: http://dx.doi.org/10.1016/j.biortech.2012.12.081
Fan L, Vonshak A, Boussiba S. Effect of temperature and irradiance on growth of Haematococcus pluvialis (Chlorophyceae). J Phycol. 1994;30:829–33.
Sarada R, Tripathi U, Ravishankar GA. Influence of stress on astaxanthin production in Haematococcus pluvialis grown under different culture conditions. Process Biochem. 2002;37(6):623–7.
Huntley ME, Redalje DG. CO2 mitigation and renewable oil from photosynthetic microbes: A new appraisal. Vol. 12, Mitigation and Adaptation Strategies for Global Change. 2007. 573–608 p.
Bitaubé Pérez E, Caro Pina I, Pérez Rodríguez L. Kinetic model for growth of Phaeodactylum tricornutum in intensive culture photobioreactor. Biochem Eng J. 2008;40(3):520–5.
Kebede E, Ahlgren G. Optimum growth conditions and light utilization efficiency of Spirulina platensis (= Arthrospira fusiformis) (Cyanophyta) from Lake Chitu, Ethiopia. Hydrobiologia. 1996;332(2):99–109.
De Oliveira MACL, Monteiro MPC, Robbs PG, Leite SGF. Growth and chemical composition of Spirulina maxima and Spirulina platensis biomass at different temperatures. Aquac Int. 1999;7(4):261–75.
Colla LM, Oliveira Reinehr C, Reichert C, Costa JAV. Production of biomass and nutraceutical compounds by Spirulina platensis under different temperature and nitrogen regimes. Bioresour Technol. 2007;98(7):1489–93.
Kumar A, Yuan X, Sahu AK, Ergas SJ, Van Langenhove H, Dewulf J. A hollow fiber membrane photo-bioreactor for CO2 sequestration from combustion gas coupled with wastewater treatment: A process engineering approach. J Chem Technol Biotechnol. 2010;85(3):387–94.
Nakano Y, Miyatake K, Okuno H, Hamazaki K, Takenaka S, Honami N, et al. GROWTH OF PHOTOSYNTHETIC ALGAE EUGLENA IN HIGH CO2 CONDITIONS AND ITS PHOTOSYNTHETIC CHARACTERISTICS. Acta Hortic [Internet]. 1996;49–54. Available from: https://www.ishs.org/ishs-article/440_9#:~:text=Acta Horticulturae 440-,GROWTH OF PHOTOSYNTHETIC ALGAE EUGLENA IN HIGH,CONDITIONS AND ITS PHOTOSYNTHETIC CHARACTERISTICS&text=Photoautotrophic growth of Euglena gracilis,than 45 %25 CO2 concentration.
Kitaya Y, Azuma H, Kiyota M. Effects of temperature, CO 2 /O 2 concentrations and light intensity on cellular multiplication of microalgae, Euglena gracilis. Adv Sp Res. 2005;35(9 SPEC. ISS.):1584–8.
Benemann JR. Utilization of carbon dioxide from fossil fuel-burning power plants with biological systems. Energy Convers Manag [Internet]. 1993;34(9–11):999–1004. Available from: https://www.sciencedirect.com/science/article/abs/pii/019689049390047E#:~:text=Biological processes for CO2,gases in a practical process.
Nobutaka H, Toshifumi Takeuchia, Fukuju Y, J.Barnesa D, ISao Karubea. Tolerance of microalgae to high CO2 and high temperature. Phytochemistry [Internet]. 1992;10(31):3345–8. Available from: https://www.sciencedirect.com/science/article/abs/pii/003194229283682O#:~:text=Scenedesmus was better able to,able to tolerate elevated temperatures.
Graham LKE, Wilcox LW. The origin of alternation of generations in land plants: A focus on matrotrophy and hexose transport. Philos Trans R Soc B Biol Sci. 2000;355(1398):757–67.
Anguselvi V, Masto RE, Mukherjee A, Singh PK. CO2 Capture for Industries by Algae. In: Wong YK, editor. Algae [Internet]. London: IntechOpen; 2019. Available from: https://www.intechopen.com/books/algae/co-sub-2-sub-capture-for-industries-by-algae
Brown LM. Uptake of carbon dioxide from flue gas by microalgae. Energy Convers Manag. 1996;37(6–8):1363–7.
Sobczuk TM, Camacho FG, Rubio FC, Fernández FGA, Grima EM. Carbon dioxide uptake efficiency by outdoor microalgal cultures in tubular airlift photobioreactors. Biotechnol Bioeng [Internet]. 2000;67(4):465–75. Available from: https://onlinelibrary.wiley.com/doi/10.1002/(SICI)1097-0290(20000220)67:4%3C465::AID-BIT10%3E3.0.CO;2-9
Lee YK, Hing HK. Supplying CO2 to photosynthetic algal cultures by diffusion through gas-permeable membranes. Appl Microbiol Biotechnol. 1989;31(3):298–301.
Benemann JR, Tillett DM, Weissman JC. Microalgae biotechnology. Trends Biotechnol. 1987;5(2):47–53.
Oswald JA. Large-Scale Algal Culture Systems (Engineering Aspects). In: Borowitzka LJ, Borowitzka MA, editors. Microalgal biotechnology [Internet]. Cambridge: Cambridge University Press; 1988. p. 357–95. Available from: https://books.google.co.in/books/about/Micro_algal_Biotechnology.html?id=FpprQgAACAAJ
Tapie P, Bernard A. Microalgae production: Technical and economic evaluations. Biotechnol Bioeng. 1988;32(7):873–85.
Papazi A, Makridis P, Divanach P, Kotzabasis K. Bioenergetic changes in the microalgal photosynthetic apparatus by extremely high CO2 concentrations induce an intense biomass production. Physiol Plant [Internet]. 2008;132(3):338–49. Available from: https://onlinelibrary.wiley.com/doi/abs/10.1111/j.1399-3054.2007.01015.x
Hessen DO, Anderson TR. Excess carbon in aquatic organisms and ecosystems: Physiological, ecological, and evolutionary implications. Limnol Oceanogr. 2008;53(4):1685–96.
Tsuzuki M, Gantar M, Aizawa K, Miyachi S. Ultrastructure of Dunaliella tertiolecta Cells Grown under Low and High CO2 Concentrations. Plant Cell Phys [Internet]. 1986;27(4):737–9. Available from: https://academic.oup.com/pcp/article-abstract/27/4/737/1850783?redirectedFrom=fulltext
Calvin M. Forty years of photosynthesis and related activities. Photosynth Res [Internet]. 1989;21(1):3–16. Available from: https://link.springer.com/article/10.1007/BF00047170
Ho SH, Chen CY, Lee DJ, Chang JS. Perspectives on microalgal CO2-emission mitigation systems - A review. Biotechnol Adv [Internet]. 2011;29(2):189–98. Available from: http://dx.doi.org/10.1016/j.biotechadv.2010.11.001
?ervený J, Šetlík I, Trtílek M, Nedbal L. Photobioreactor for cultivation and real-time, in-situ measurement of O2 and CO2 exchange rates, growth dynamics, and of chlorophyll fluorescence emission of photoautotrophic microorganisms. Eng Life Sci. 2009;9(3):247–53.
Cohen Y, Nisnevitch M, Anker Y. Innovative large-scale photobioreactor for coal propelled power plant effluents treatment. Algal Res [Internet]. 2020;52(September):102101. Available from: https://doi.org/10.1016/j.algal.2020.102101
Yang C, Hua Q, Shimizu K. Energetics and carbon metabolism during growth of microalgal cells under photoautotrophic, mixotrophic and cyclic light-autotrophic/dark-heterotrophic conditions. Biochem Eng J. 2000;6(2):87–102.
Grobbelaar JU, Nedbal L, Tichý V. Influence of high frequency light/dark fluctuations on photosynthetic characteristics of microalgae photoacclimated to different light intensities and implications for mass algal cultivation. J Appl Phycol. 1996;8(4–5):335–43.
Jacob-Lopes E, Gimenes Scoparo CH, Queiroz MI, Franco TT. Biotransformations of carbon dioxide in photobioreactors. Energy Convers Manag [Internet]. 2010;51(5):894–900. Available from: http://dx.doi.org/10.1016/j.enconman.2009.11.027
You T, Barnett SM. Effect of light quality on production of extracellular polysaccharides and growth rate of Porphyridium cruentum. Biochem Eng J. 2004;19(3):251–8.
Ravelonandro PH, Ratianarivo DH, Joannis?Cassan C, Isambert A, Raherimandimby M. Influence of light quality and intensity in the cultivation of Spirulina platensis from Toliara (Madagascar) in a closed system. J Chem Technol Biotechnol [Internet]. 2008;83(6):842–8. Available from: https://onlinelibrary.wiley.com/doi/abs/10.1002/jctb.1878
Rosgaard L, de Porcellinis AJ, Jacobsen JH, Frigaard NU, Sakuragi Y. Bioengineering of carbon fixation, biofuels, and biochemicals in cyanobacteria and plants. J Biotechnol [Internet]. 2012;162(1):134–47. Available from: http://dx.doi.org/10.1016/j.jbiotec.2012.05.006
Gimpel JA, Specht EA, Georgianna DR, Mayfield SP. Advances in microalgae engineering and synthetic biology applications for biofuel production. Curr Opin Chem Biol [Internet]. 2013;17(3):489–95. Available from: http://dx.doi.org/10.1016/j.cbpa.2013.03.038
Chang RL, Ghamsari L, Manichaikul A, Hom EFY, Balaji S, Fu W, et al. Metabolic network reconstruction of Chlamydomonas offers insight into light-driven algal metabolism. Mol Syst Biol. 2011;7(518).
FlaviaWinck V, DavidPáez Melo O, DiegoRiaño-Pachón M, MarinaMartins CM, Caldana C, AndrésGonzález Barrios F. Analysis of sensitive CO2 pathways and genes related to carbon uptake and accumulation in chlamydomonas reinhardtii through genomic scale modeling and experimental validation. Front Plant Sci. 2016;7(FEB2016):1–12.
Tsoutsos T, Kouloumpis V, Zafiris T, Foteinis S. Life Cycle Assessment for biodiesel production under Greek climate conditions. J Clean Prod [Internet]. 2010;18(4):328–35. Available from: http://dx.doi.org/10.1016/j.jclepro.2009.11.002
Gnansounou E, Dauriat A, Villegas J, Panichelli L. Life cycle assessment of biofuels: Energy and greenhouse gas balances. Bioresour Technol [Internet]. 2009;100(21):4919–30. Available from: http://dx.doi.org/10.1016/j.biortech.2009.05.067
Khoo HH, Sharratt PN, Das P, Balasubramanian RK, Naraharisetti PK, Shaik S. Life cycle energy and CO2 analysis of microalgae-to-biodiesel: Preliminary results and comparisons. Bioresour Technol [Internet]. 2011;102(10):5800–7. Available from: http://dx.doi.org/10.1016/j.biortech.2011.02.055
Campbell PK, Beer T, Batten D. Life cycle assessment of biodiesel production from microalgae in ponds. Bioresour Technol [Internet]. 2011;102(1):50–6. Available from: http://dx.doi.org/10.1016/j.biortech.2010.06.048
Kadam KL. Microalgae Production from Power Plant Flue Gas: Environmental Implications on a Life Cycle Basis [Internet]. Contract. 2001. Available from: www.nrel.gov/docs/fy01osti/29417.pdf
Alabi AO, Tampier M BE. Microalgae Technogies and Processes for Biofuels/ Bioenergy Production in British Columbia. [Internet]. Canada; 2009. Available from: https://www.etipbioenergy.eu/databases/reports/69-microalgae-technologies-processes-for-biofuels-bioenergy-production-in-british-columbia-current-technology-barriers-to-implementation
Habib MAB, Parvin M, Huntington TC, Hasan MR. A REVIEW ON CULTURE, PRODUCTION AND USE OF SPIRULINA AS FOOD FOR HUMANS AND FEEDS FOR DOMESTIC ANIMALS AND FISH [Internet]. FAO Fisheries and Aquaculture Circular. No. 1034. Rome; 2008. Available from: http://www.fao.org/3/i0424e/i0424e00.htm
Becker EW. Microalgae for Human and Animal Nutrition. In: Richmond A, editor. Handbook of Microalgal Culture: Biotechnology and Applied Phycology [Internet]. State Avenue, Ames, Iowa, USA: a Blackwell Publishing Company; 2013. p. 312–51. Available from: http://dl.icdst.org/pdfs/files/8a0e128e46252f218192dc8d9cfa090e.pdf
Todd Lorenz R, Cysewsk GR. Commercial potential for Haematococcus microalgae as a natural source of astaxanthin. Trends Biotechnol [Internet]. 2000;18(4):160-167. Available from: https://www.sciencedirect.com/science/article/abs/pii/S0167779900014335
Sekar S, Chandramohan M. Phycobiliproteins as a commodity: Trends in applied research, patents and commercialization. J Appl Phycol. 2008;20(2):113–36.
Merchant SS, Prochnik SE, Vallon O, Harris EH, Sanderfoot SA, Spalding MH, et al. The Chlamydomonas Genome Reveals the Evolution of Key. Natl institutes Heal. 2007;318(5848):245–50.
Matsuo T, Ishiura M. Chlamydomonas reinhardtii as a new model system for studying the molecular basis of the circadian clock. FEBS Lett. 2011;585(10):1495–502.
Hermsmeier D, Schulz R, Senger H. Formation of light-harvesting complexes of photosystem II in Scenedesmus - II. Different patterns of light-regulation of Lhc-gene expression in green and greening cells. Planta. 1994;193(3):406–12.
Yamano T, Fukuzawa H. Carbon-concentrating mechanism in a green alga, Chlamydomonas reinhardtii, revealed by transcriptome analyses. J Basic Microbiol. 2009;49(1):42–51.
Rolland N, Atteia A, Decottignies P, Garin J, Hippler M, Kreimer G, et al. Chlamydomonas proteomics. Curr Opin Microbiol. 2009;12(3):285–91.
Mühlhaus T, Weiss J, Hemme D, Sommer F, Schroda M. Quantitative shotgun proteomics using a uniform 15N-labeled standard to monitor proteome dynamics in time course experiments reveals new insights into the heat stress response of Chlamydomonas reinhardtii. Mol Cell Proteomics. 2011;10(9):1–27.
Cadoret JP, Garnier M, Saint-Jean B. Microalgae, Functional Genomics and Biotechnology. Vol. 64, Advances in Botanical Research. 2012. 285–341 p.
Raven JA. Inorganic Carbon Acquisition by Marine Autotrophs. Adv Bot Res [Internet]. 1997;27:85–209. Available from: https://www.sciencedirect.com/science/article/pii/S0065229608602815
Moroney J V., Somanchi A. How do algae concentrate CO2 to increase the efficiency of photosynthetic carbon fixation? Plant Physiol. 1999;119(1):9–16.
Price GD, Coleman JR, Badger MR. Association of carbonic anhydrase activity with carboxysomes isolated from the cyanobacterium Synechococcus PCC7942. Plant Physiol. 1992;100(2):784–93.
Meyer MT, Itakura AK, Patena W, Wang L, He S, Emrich-Mills T, et al. Assembly of the algal CO2-fixing organelle, the pyrenoid, is guided by a Rubisco-binding motif. bioRxiv. 2020;(November):1–11.
Yang B, Liu J, Ma X, Guo B, Liu B, Wu T, et al. Genetic engineering of the Calvin cycle toward enhanced photosynthetic CO2 fixation in microalgae. Biotechnol Biofuels. 2017;10(1):1–13.
Fang L, Lin HX, Low CS, Wu MH, Chow Y, Lee YK. Expression of the Chlamydomonas reinhardtii Sedoheptulose-1,7-bisphosphatase in Dunaliella bardawil leads to enhanced photosynthesis and increased glycerol production. Plant Biotechnol J. 2012;10(9):1129–35.
Moroney J V., Ma Y, Frey WD, Fusilier KA, Pham TT, Simms TA, et al. The carbonic anhydrase isoforms of Chlamydomonas reinhardtii: Intracellular location, expression, and physiological roles. Photosynth Res. 2011;109(1–3):133–49.
Yamano T, Sato E, Iguchi H, Fukuda Y, Fukuzawa H. Characterization of cooperative bicarbonate uptake into chloroplast stroma in the green alga Chlamydomonas reinhardtii. Proc Natl Acad Sci U S A. 2015;112(23):7315–20.
Gee CW, Niyogi KK. The carbonic anhydrase CAH1 is an essential component of the carbon-concentrating mechanism in Nannochloropsis oceanica. Proc Natl Acad Sci U S A. 2017;114(17):4537–42.
Khandavalli LVNS, Lodha T, Abdullah M, Guruprasad L, Chintalapati S, Chintalapati VR. Insights into the carbonic anhydrases and autotrophic carbon dioxide fixation pathways of high CO2 tolerant Rhodovulum viride JA756. Microbiol Res [Internet]. 2018;215(July):130–40. Available from: https://doi.org/10.1016/j.micres.2018.07.006
Fujiwara S, Fukuzawa H, Tachiki A. Structure and differential expression of two genes encoding carbonic anhydrase in Chlamydomonas reinhardtii. Proc Nati Acad Sci USA [Internet]. 1990;87(December):9779–83. Available from: https://pubmed.ncbi.nlm.nih.gov/2124702/
Moroney J V., Husic HD, Tolbert NE. Effect of Carbonic Anhydrase Inhibitors on Inorganic Carbon Accumulation by Chlamydomonas reinhardtii . Plant Physiol. 1985;79(1):177–83.
Winck FV, Arvidsson S, Riaño-Pachón DM, Hempe S, Koseska A, Nikoloski Z, et al. Genome-wide identification of regulatory elements and reconstruction of gene regulatory networks of the green alga Chlamydomonas reinhardtii under carbon deprivation. PLoS One. 2013;8(11):1–16.
Kumar G, Shekh A, Jakhu S, Sharma Y, Kapoor R, Sharma TR. Bioengineering of Microalgae: Recent Advances, Perspectives, and Regulatory Challenges for Industrial Application. Front Bioeng Biotechnol. 2020;8(September).
Winck F V, Melo DOP, Riaño-pachón DM, Martins MCM. Analysis of Sensitive CO 2 Pathways and Genes Related to Carbon Uptake and Accumulation in Chlamydomonas reinhardtii through Genomic Scale Modeling and Experimental Validation. 2016;7(February):1–12.
Jungnick N, Ma Y, Mukherjee B, Cronan JC, Speed DJ, Laborde SM, et al. The carbon concentrating mechanism in Chlamydomonas reinhardtii: Finding the missing pieces. Photosynth Res. 2014;121(2–3):159–73.
Park Y Il, Karlsson J, Rojdestvenski I, Pronina N, Klimov V, Öquist G, et al. Role of a novel photosystem II-associated carbonic anhydrase in photosynthetic carbon assimilation in Chlamydomonas reinhardtii. FEBS Lett. 1999;444(1):102–5.
Villarejo A, Shutova T, Moskvin O, Forssén M, Klimov V V., Samuelsson G. A photosystem II-associated carbonic anhydrase regulates the efficiency of photosynthetic oxygen evolution. EMBO J. 2002;21(8):1930–8.
Spalding MH. Microalgal carbon-dioxide-concentrating mechanisms: Chlamydomonas inorganic carbon transporters. J Exp Bot. 2008;59(7):1463–73.
Kuo CM, Lin TH, Yang YC, Zhang WX, Lai JT, Wu HT, et al. Ability of an alkali-tolerant mutant strain of the microalga Chlorella sp. AT1 to capture carbon dioxide for increasing carbon dioxide utilization efficiency. Bioresour Technol [Internet]. 2017;244:243–51. Available from: http://dx.doi.org/10.1016/j.biortech.2017.07.096
Fu W, Nelson DR, Mystikou A, Daakour S, Salehi-Ashtiani K. Advances in microalgal research and engineering development. Curr Opin Biotechnol [Internet]. 2019;59:157–64. Available from: https://doi.org/10.1016/j.copbio.2019.05.013
Vandepoele K, Van Bel M, Richard G, Van Landeghem S, Verhelst B, Moreau H, et al. pico-PLAZA, a genome database of microbial photosynthetic eukaryotes. Environ Microbiol. 2013;15(8):2147–53.
Zheng H, Chien C, Hsu BJ, Liu T, Chen CN, Chang W. AlgaePath?: comprehensive analysis of metabolic pathways using transcript abundance data from next-generation sequencing in green algae. BMC Genomics [Internet]. 2014;15(196):1–12. Available from: https://bmcgenomics.biomedcentral.com/articles/10.1186/1471-2164-15-196
Aoki Y, Okamura Y, Ohta H, Kinoshita K, Obayashi T. ALCOdb: Gene coexpression database for microalgae. Plant Cell Physiol. 2016;57(1):e3.
Bae S, Park J, Kim JS. Cas-OFFinder: A fast and versatile algorithm that searches for potential off-target sites of Cas9 RNA-guided endonucleases. Bioinformatics. 2014;30(10):1473–5.
Lei Y, Lu L, Liu HY, Li S, Xing F, Chen LL. CRISPR-P: A web tool for synthetic single-guide RNA design of CRISPR-system in plants. Mol Plant. 2014;7(9):1494–6.
Liu H, Ding Y, Zhou Y, Jin W, Xie K, Chen LL. CRISPR-P 2.0: An Improved CRISPR-Cas9 Tool for Genome Editing in Plants. Mol Plant [Internet]. 2017;10(3):530–2. Available from: http://dx.doi.org/10.1016/j.molp.2017.01.003
Liu T, Pan S, Li Y, She Q. Type III CRISPR-Cas System: Introduction And Its Application for Genetic Manipulations. Curr Issues Mol Biol [Internet]. 2017;26:1–14. Available from: https://europepmc.org/article/med/28879852
Park J, Bae S, Kim JS. Cas-Designer: A web-based tool for choice of CRISPR-Cas9 target sites. Bioinformatics. 2015;31(24):4014–6.
Stemmer M, Thumberger T, Del Sol Keyer M, Wittbrodt J, Mateo JL. CCTop: An intuitive, flexible and reliable CRISPR/Cas9 target prediction tool. PLoS One. 2015;10(4):1–11.
Labun K, Montague TG, Gagnon JA, Thyme SB, Valen E. CHOPCHOP v2: a web tool for the next generation of CRISPR genome engineering. Nucleic Acids Res. 2016;44(W1):W272–6.
Montague TG, Cruz JM, Gagnon JA, Church GM, Valen E. CHOPCHOP: A CRISPR/Cas9 and TALEN web tool for genome editing. Nucleic Acids Res. 2014;42(W1):1–7.
Doench JG, Fusi N, Sullender M, Hegde M, Vaimberg EW, Donovan KF, et al. Optimized sgRNA design to maximize activity and minimize off-target effects of CRISPR-Cas9. Nat Biotechnol [Internet]. 2016;34(2):184–91. Available from: http://dx.doi.org/10.1038/nbt.3437
Park J, Lim K, Kim JS, Bae S. Cas-analyzer: An online tool for assessing genome editing results using NGS data. Bioinformatics. 2017;33(2):286–8.
Kim D, Bae S, Park J, Kim E, Kim S, Yu HR, et al. Digenome-seq: Genome-wide profiling of CRISPR-Cas9 off-target effects in human cells. Nat Methods [Internet]. 2015;12(3):237–43. Available from: http://dx.doi.org/10.1038/nmeth.3284
Tanwar A, Sharma S, Kumar S. Targeted genome editing in algae using CRISPR/Cas9. Indian J Plant Physiol [Internet]. 2018;23(4):653–69. Available from: https://doi.org/10.1007/s40502-018-0423-3
Grossman AR. Paths toward algal genomics. Plant Physiol. 2005;137(2):410–27.
Tettelin H, Masignani V, Cieslewicz MJ, Donati C, Medini D, Ward NL, et al. Erratum: Genome analysis of multiple pathogenic isolates of Streptococcus agalactiae: Implications for the microbial “pan-genome” (Proceedings of the National Academy of Sciences of the United States of America (September 27, 2005) 102, 39 (13950-13955)). Proc Natl Acad Sci U S A. 2005;102(45):16530.
Du YC, Peddi SR, Spreitzer RJ. Assessment of structural and functional divergence far from the large subunit active site of ribulose-1,5-bisphosphate carboxylase/oxygenase. J Biol Chem. 2003;278(49):49401–5.
Spreitzer RJ, Peddi SR, Satagopan S. Phylogenetic engineering at an interface between large and small subunits imparts land-plant kinetic properties to algal Rubisco. Proc Natl Acad Sci U S A. 2005;102(47):17225–30.
Blaby-Haas CE, Merchant SS. Comparative and Functional Algal Genomics. Annu Rev Plant Biol. 2019;70(August):605–38.
Radakovits R, Jinkerson RE, Darzins A, Posewitz MC. Genetic engineering of algae for enhanced biofuel production. Eukaryot Cell. 2010;9(4):486–501.
Liolios K, Mavromatis K, Tavernarakis N, Kyrpides NC. The Genomes On Line Database (GOLD) in 2007: Status of genomic and metagenomic projects and their associated metadata. Nucleic Acids Res. 2008;36(SUPPL. 1):475–9.
Wang Q, Lu Y, Xin Y, Wei L, Huang S, Xu J. Genome editing of model oleaginous microalgae Nannochloropsis spp. by CRISPR/Cas9. Plant J. 2016;88(6):1071–81.
Fulke A, Chakrabarti T, Kannan K. CO2 Sequestration by Microalgae: Advances and Perspectives. In: Vasconcelos JR, Gerken H, Liu J, Sun Z, editors. Recent Advances in Microalgal Biotechnology [Internet]. OMICS International; 2016. Available from: https://www.esciencecentral.org/ebooks/ebookdetail/recent-advances-in-microalgal-biotechnology
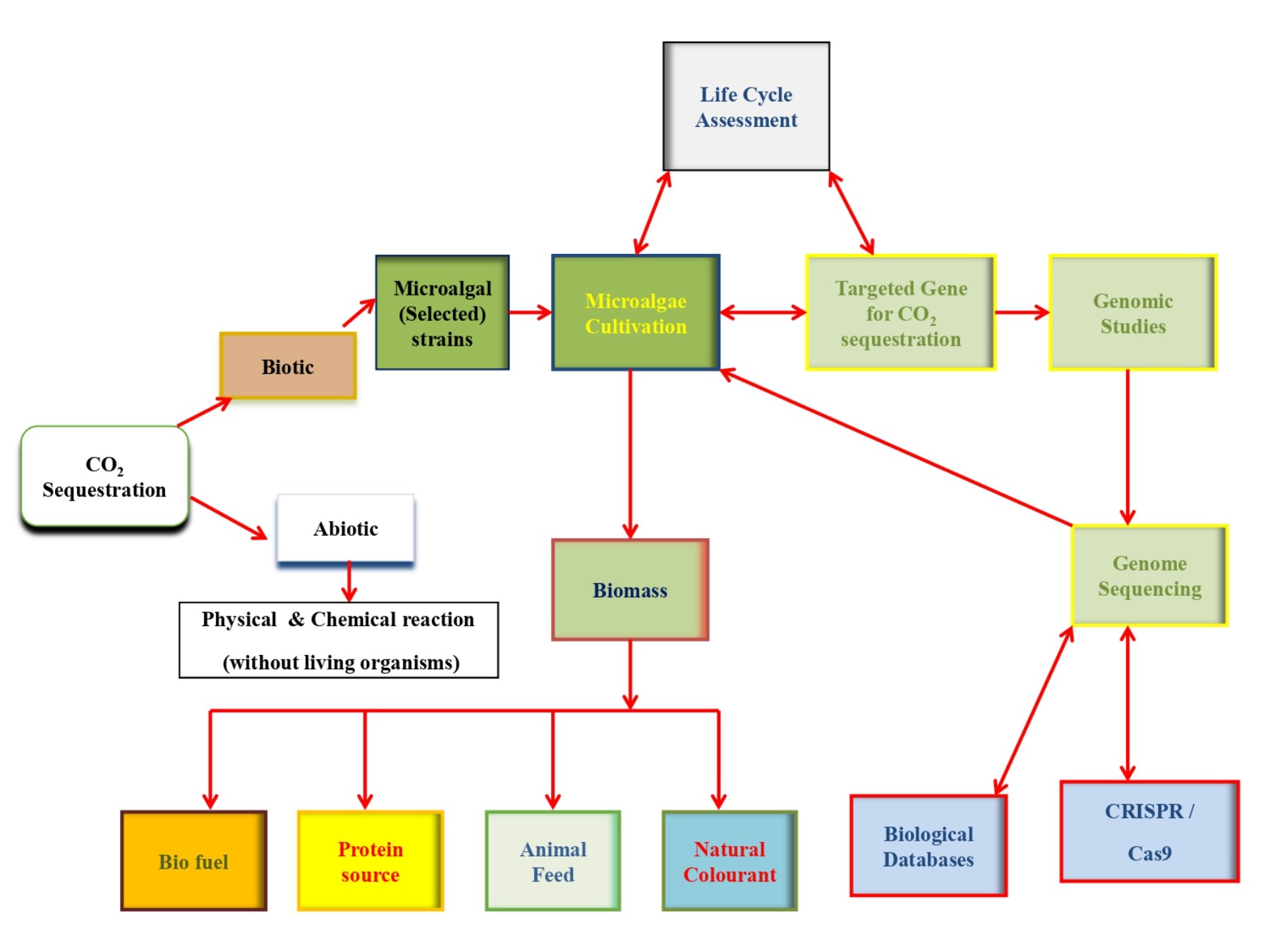
Downloads
Published
Versions
- 01-04-2022 (2)
- 13-03-2022 (1)
How to Cite
Issue
Section
License
Copyright (c) 2021 R Ranjith Kumar, Monika S Jency, B Bhavatarini, M. Jeslyn Priscilla Devapriya

This work is licensed under a Creative Commons Attribution 4.0 International License.
Copyright and Licence details of published articles
Authors who publish with this journal agree to the following terms:
- Authors retain copyright and grant the journal right of first publication with the work simultaneously licensed under a Creative Commons Attribution License that allows others to share the work with an acknowledgement of the work's authorship and initial publication in this journal.
- Authors are able to enter into separate, additional contractual arrangements for the non-exclusive distribution of the journal's published version of the work (e.g., post it to an institutional repository or publish it in a book), with an acknowledgement of its initial publication in this journal.
Open Access Policy
Plant Science Today is an open access journal. There is no registration required to read any article. All published articles are distributed under the terms of the Creative Commons Attribution License (CC Attribution 4.0), which permits unrestricted use, distribution, and reproduction in any medium, provided the original author and source are credited (https://creativecommons.org/licenses/by/4.0/). Authors are permitted and encouraged to post their work online (e.g., in institutional repositories or on their website) prior to and during the submission process, as it can lead to productive exchanges, as well as earlier and greater citation of published work (See The Effect of Open Access).