Role of microbes in alleviating abiotic stress in plants
DOI:
https://doi.org/10.14719/pst.2215Keywords:
Abiotic stress, Bacteria, Fungi, Mitigation, Plant microbe interactionAbstract
The leading threat to agricultural productivity is the recurrent variations in environmental conditions. A battery of abiotic stresses namely flooding, salinity, temperature, drought, heavy metal toxicities, nutrient deficiencies and oxidative stress causes irreversible damage resulting in loss of plant’s vigor and yield. The relationship between plants and microorganisms is a highly dynamic system. The plant microbiome consists of plant growth-promoting bacteria and fungi. In the last decade, many microbes that give hosts the ability to withstand abiotic stress have been characterized in detail. Their beneficial association with plants enables the plant to endure different stresses imposed on them thereby enhancing the plant's sustainability and productivity. For sustainable agriculture, it is very significant to comprehend microbiome-assisted mechanisms for mitigating abiotic stress. This review will shed light on the current knowledge about the roles of various microorganisms in mitigating against abiotic stresses. The understanding of these mechanisms will help to increase the yield of plants and meet the food demands of the expanding population.
Downloads
References
Zaidi SSA, Mahas A, Vanderschuren H, Mahfouz MM. Engineering crops of the future: CRISPR approaches to develop climate-resilient and disease-resistant plants. Genome Biol. 2020;21:289. https://doi.org/10.1186/s13059-020-02204-y.
Habib-ur-Rahman M, Ahmad A, Raza A et al. Impact of climate change on agricultural production; Issues, challenges and opportunities in Asia. Front Plant Sci. 2022;13. https://doi.org/10.3389/fpls.2022.925548.
Ranjha MMAN, Shafique B, Khalid W, Nadeem HR, Mueen-ud-Din G, Khalid MZ. Applications of biotechnology in food and agriculture: A mini-review. Proc Natl Acad Sci India Sect B Biol Sci. 2022;92:11-15. https://doi.org/10.1007/s40011-021-01320-4.
Phillips T. Genetically modified organisms (GMOs): Transgenic crops and recombinant DNA technology. Nat Educ. 2008;1:213.
Liu W, Yuan JS, Stewart Jr CN. Advanced genetic tools for plant biotechnology. Nat Rev Genet. 2013;14:781-93. https://doi.org/10.1038/nrg3583.
Gimenez E, Salinas M, Manzano-Agugliaro F. Worldwide research on plant defense against biotic stresses as improvement for sustainable agriculture. Sustainability. 2018;10:391. https://doi.org/10.3390/su10020391.
He M, He CQ, Ding NZ. Abiotic stresses: General defenses of land plants and chances for engineering multistress tolerance. Front Plant Sci. 2018;9. https://doi.org/10.3389/fpls.2018.01771
Grayson M. Agriculture and drought. Nature. 2013;501:S1-S1. https://doi.org/10.1038/501S1a.
Grover M, Ali SZ, Sandhya V, Rasul A, Venkateswarlu B. Role of microorganisms in adaptation of agriculture crops to abiotic stresses. World J Microbiol Biotechnol. 2011;27:1231-40. https://doi.org/10.1007/s11274-010-0572-7.
Venkateswarlu B, Shankar AK, Gogoi AK. Climate change adaptation and mitigation in Indian agriculture. In: Rao GP editor. Climate change adaptation strategies in agriculture and allied sectors. Scientific Publishers India. 2011; p. 85-95.
Inbaraj MP. Plant-microbe interactions in alleviating abiotic stress—A mini review. Front Agron. 2021;3. https://doi.org/10.3389/fagro.2021.667903.
Santos LF, Olivares FL. Plant microbiome structure and benefits for sustainable agriculture. Curr Plant Biol. 2021;26:100198. https://doi.org/10.1016/j.cpb.2021.100198.
Khan A, Sayyed RZ, Seifi S. Rhizobacteria: Legendary soil guards in abiotic stress management. In: Sayyed R, Arora N, Reddy M, editor. Plant Growth Promoting Rhizobacteria for Sustainable Stress Management. Microorganisms for Sustainability. Singapore: Springer; 2019. p. 327-43. https://doi.org/10.1007/978-981-13-6536-2_15.
Kour D, Rana KL, Yadav N et al. Rhizospheric microbiomes: Biodiversity, mechanisms of plant growth promotion and biotechnological applications for sustainable agriculture. In: Kumar A, Meena V editor. Plant growth promoting rhizobacteria for agricultural sustainability. Singapore: Springer Singapore. 2019; p. 19-65. https://doi.org/10.1007/978-981-13-7553-8_2.
Bhattacharyya PN, Jha DK. Plant growth-promoting rhizobacteria (PGPR): emergence in agriculture. World J Microbiol Biotechnol. 2012;28:1327-50. https://doi.org/10.1007/s11274-011-0979-9.
Santoyo G, Urtis-Flores CA, Loeza-Lara PD, Orozco-Mosqueda M del C, Glick BR. Rhizosphere colonization determinants by plant growth-promoting rhizobacteria (PGPR). Biology (Basel). 2021;10:475. https://doi.org/10.3390/biology10060475.
Philippot L, Raaijmakers JM, Lemanceau P, van der Putten WH. Going back to the roots: the microbial ecology of the rhizosphere. Nat Rev Microbiol. 2013;11:789-99. https://doi.org/10.1038/nrmicro3109.
Santos ML dos, Berlitz DL, Wiest SLF, Schünemann R, Knaak N, Fiuza LM. Benefits associated with the interaction of endophytic bacteria and plants. Brazilian Arch Biol Technol. 2018;61. https://doi.org/10.1590/1678-4324-2018160431.
El Enshasy HA, Ambehabati KK, El Baz AF et al. Trichoderma: Biocontrol agents for promoting plant growth and soil health. In: Yadav A, Mishra S, Kour D, Yadav NKA editor. Agriculturally important fungi for sustainable agriculture. Functional Annotation for Crop Protection. Cham: Springer. 2020; p. 239-59. https://doi.org/10.1007/978-3-030-48474-3_8.
Singh N, Singh A, Dahiya P. Plant growth-promoting endophytic fungi from different habitats and their potential applications in agriculture. In: Yadav AN editor. Recent Trends in Mycological Research. Fungal Biology. Cham: Springer. 2021;p.69-87. https://doi.org/10.1007/978-3-030-60659-6_3.
Rustamova N, Litao N, Bozorov K, Sayyed R, Aisa HA YA. Plant-associated endophytic fungi: A source of structurally diverse and bioactive natural products. Plant Cell Biotechnol Mol Biol. 2022;23:1-19.
Sahoo S, Sarangi S, Kerry RG. Bioprospecting of endophytes for agricultural and environmental sustainability. In: Patra JK editor. Microbial Biotechnology. Singapore: Springer. 2017; p. 429-58. https://doi.org/10.1007/978-981-10-6847-8_19.
Gill SS, Gill R, Trivedi DK et al. Piriformospora indica: Potential and significance in plant stress tolerance. Front Microbiol. 2016;7. https://doi.org/10.3389/fmicb.2016.00332.
Diagne N, Ngom M, Djighaly PI, Fall D, Hocher V, Svistoonoff S. Roles of arbuscular mycorrhizal fungi on plant growth and performance: Importance in biotic and abiotic stressed regulation. Diversity. 2020;12:370. https://doi.org/10.3390/d12100370.
Begum N, Qin C, Ahanger MA et al. Role of arbuscular mycorrhizal fungi in plant growth regulation: Implications in abiotic stress tolerance. Front Plant Sci. 2019;10. https://doi.org/10.3389/fpls.2019.01068.
Kashyap PL, Rai P, Srivastava AK, Kumar S. Trichoderma for climate resilient agriculture. World J Microbiol Biotechnol. 2017;33:155. https://doi.org/10.1007/s11274-017-2319-1.
Gopalakrishnan S, Sathya A, Vijayabharathi R, Varshney RK, Gowda CLL, Krishnamurthy L. Plant growth promoting rhizobia: challenges and opportunities. 3 Biotech. 2015;5:355-77. https://doi.org/10.1007/s13205-014-0241-x.
Koza N, Adedayo A, Babalola O, Kappo A. Microorganisms in plant growth and development: Roles in abiotic stress tolerance and secondary metabolites secretion. Microorganisms. 2022;10:1528. https://doi.org/10.3390/microorganisms10081528.
Marothia D, Kaur N, Kumar Pati P. Abiotic stress responses in plants: Current knowledge and future prospects. In: Fahad S, Saud S, Chen Y, Wu C, Wang D editor. Abiotic Stress in Plants. London: IntechOpen; 2021. https://doi.org/10.5772/intechopen.93824.
Zhang H, Zhu J, Gong Z, Zhu JK. Abiotic stress responses in plants. Nat Rev Genet. 2022;23:104-19. https://doi.org/10.1038/s41576-021-00413-0.
Waadt R, Seller CA, Hsu P-K, Takahashi Y, Munemasa S, Schroeder JI. Plant hormone regulation of abiotic stress responses. Nat Rev Mol Cell Biol. 2022;23:680-94. https://doi.org/10.1038/s41580-022-00479-6.
Abdul Rahman NSN, Abdul Hamid NW, Nadarajah K. Effects of Abiotic Stress on Soil Microbiome. Int J Mol Sci. 2021;22:9036. https://doi.org/10.3390/ijms22169036.
Ameh T, Sayes CM. The potential exposure and hazards of copper nanoparticles: A review. Environ Toxicol Pharmacol. 2019;71:103220. https://doi.org/10.1016/j.etap.2019.103220.
Narsing Rao MP, Dong ZY, Xiao M, Li WJ. Effect of salt stress on plants and role of microbes in promoting plant growth under salt stress. In: Giri B, Varma A editor. Microorganisms in saline environments: Strategies and functions. Soil Biology. Cham: Springer. 2019; p. 423-35. https://doi.org/10.1007/978-3-030-18975-4_18.
Uçarli C. Effects of salinity on seed germination and early seedling stage. In: Fahad S, Saud S, Chen Y, Wu C, Wang D editor. Abiotic stress in plants. London: IntechOpen; 2021. https://doi.org/10.5772/intechopen.93647.
Ketehouli T, Idrice Carther KF, Noman M, Wang FW, Li XW, Li HY. Adaptation of plants to salt stress: Characterization of Na+ and K+ transporters and role of CBL gene family in regulating salt stress response. Agronomy. 2019;9:687. https://doi.org/10.3390/agronomy9110687.
Hnilickova H, Kraus K, Vachova P, Hnilicka F. Salinity stress affects photosynthesis, malondialdehyde formation and proline content in Portulaca oleracea L. Plants. 2021;10:845. https://doi.org/10.3390/plants10050845.
Cai Z, Wang X, Bhadra S, Gao Q. Distinct factors drive the assembly of quinoa-associated microbiomes along elevation. Plant Soil. 2020;448:55-69. https://doi.org/10.1007/s11104-019-04387-1.
Silva EN, Ribeiro RV, Ferreira-Silva SL, Viégas RA, Silveira JAG. Comparative effects of salinity and water stress on photosynthesis, water relations and growth of Jatropha curcas plants. J Arid Environ. 2010;74:1130-37. https://doi.org/10.1016/j.jaridenv.2010.05.036.
Acosta-Motos J, Ortuño M, Bernal-Vicente A, Diaz-Vivancos P, Sanchez-Blanco M, Hernandez J. Plant responses to salt stress: Adaptive mechanisms. Agronomy. 2017;7:18. https://doi.org/10.3390/agronomy7010018.
Abogadallah GM. Insights into the significance of antioxidative defense under salt stress. Plant Signal Behav. 2010;5:369-74. https://doi.org/10.4161/psb.5.4.10873.
Ray RL, Fares A, Risch E. Effects of drought on crop production and cropping areas in texas. Agric Environ Lett. 2018;3:170037. https://doi.org/10.2134/ael2017.11.0037.
Orimoloye IR. Agricultural drought and its potential impacts: Enabling decision-support for food security in vulnerable regions. Front Sustain Food Syst. 2022;6. https://doi.org/10.3389/fsufs.2022.838824.
Seleiman MF, Al-Suhaibani N, Ali N et al. Drought stress impacts on plants and different approaches to alleviate its adverse effects. Plants. 2021;10:259. https://doi.org/10.3390/plants10020259.
Corso D, Delzon S, Lamarque LJ et al. Neither xylem collapse, cavitation or changing leaf conductance drive stomatal closure in wheat. Plant Cell Environ. 2020;43:854-65. https://doi.org/10.1111/pce.13722.
Yang X, Lu M, Wang Y, Wang Y, Liu Z, Chen S. Response mechanism of plants to drought stress. Horticulturae. 2021;7:50. https://doi.org/10.3390/horticulturae7030050.
Zandalinas SI, Fritschi FB, Mittler R. Global warming, climate change and environmental pollution: Recipe for a multifactorial stress combination disaster. Trends Plant Sci. 2021;26:588-99. https://doi.org/10.1016/j.tplants.2021.02.011.
Baidya A, Anwar Ali M, Atta K. Climate change and abiotic stresses in plants. In: Kimatu JN editor. Advances in plant defense mechanisms. London: IntechOpen; 2022. https://doi.org/10.5772/intechopen.105575.
Szymanska R, Slesak I, Orzechowska A, Kruk J. Physiological and biochemical responses to high light and temperature stress in plants. Environ Exp Bot. 2017;139:165-77. https://doi.org/10.1016/j.envexpbot.2017.05.002.
Bita CE, Gerats T. Plant tolerance to high temperature in a changing environment: scientific fundamentals and production of heat stress-tolerant crops. Front Plant Sci. 2013;4. https://doi.org/10.3389/fpls.2013.00273.
Hu S, Ding Y, Zhu C. Sensitivity and responses of chloroplasts to heat stress in plants. Front Plant Sci. 2020;11. https://doi.org/10.3389/fpls.2020.00375.
Diamond S, Andeer PF, Li Z et al. Mediterranean grassland soil C–N compound turnover is dependent on rainfall and depth, and is mediated by genomically divergent microorganisms. Nat Microbiol. 2019;4:1356-67. https://doi.org/10.1038/s41564-019-0449-y.
Goyal D, Yadav A, Prasad M et al. Effect of heavy metals on plant growth: An overview. In: Naeem M, Ansari A, Gill S editor Contaminants in Agriculture. Cham: Springer. 2020; p. 79-101. https://doi.org/10.1007/978-3-030-41552-5_4.
Yadav SK. Heavy metals toxicity in plants: An overview on the role of glutathione and phytochelatins in heavy metal stress tolerance of plants. South African J Bot. 2010;76:167-79. https://doi.org/10.1016/j.sajb.2009.10.007.
Deng X, Yang Y, Zeng H, Chen Y, Zeng Q. Variations in iron plaque, root morphology and metal bioavailability response to seedling establishment methods and their impacts on Cd and Pb accumulation and translocation in rice (Oryza sativa L.). J Hazard Mater. 2020;384:121343. https://doi.org/10.1016/j.jhazmat.2019.121343.
Ghori NH, Ghori T, Hayat MQ et al. Heavy metal stress and responses in plants. Int J Environ Sci Technol. 2019;16:1807-28. https://doi.org/10.1007/s13762-019-02215-8.
Kushwaha P, Kashyap PL, Kuppusamy P, Srivastava AK, Tiwari RK. Functional characterization of endophytic bacilli from pearl millet (Pennisetum glaucum) and their possible role in multiple stress tolerance. Plant Biosyst - An Int J Deal with all Asp Plant Biol. 2020;154:503-14. https://doi.org/10.1080/11263504.2019.1651773.
Raza A, Charagh S, Razzaq A, Javed R, Khan RSA, Hasanuzzaman M. Brassicaceae plants response and tolerance to drought stress: Physiological and molecular interventions. In: Hasanuzzaman M editor. The Plant Family Brassicaceae. Singapore: Springer. 2020; p. 229-61. https://doi.org/10.1007/978-981-15-6345-4_7.
Singh S, Parihar P, Singh R, Singh VP, Prasad SM. Heavy metal tolerance in plants: Role of transcriptomics, proteomics, metabolomics and ionomics. Front Plant Sci. 2016;6. https://doi.org/10.3389/fpls.2015.01143.
Chai M, Li R, Shen X, Yu L, Han J. Multiple heavy metals affect root response, iron plaque formation and metal bioaccumulation of Kandelia obovata. Sci Rep. 2022;12:14389. https://doi.org/10.1038/s41598-022-14867-7.
Thijs S, Sillen W, Weyens N, Vangronsveld J. Phytoremediation: State-of-the-art and a key role for the plant microbiome in future trends and research prospects. Int J Phytoremediation. 2017;19:23-38. https://doi.org/10.1080/15226514.2016.1216076.
Kumar B, Smita K, Cumbal Flores L. Plant mediated detoxification of mercury and lead. Arab J Chem. 2017;10:S2335-42. https://doi.org/10.1016/j.arabjc.2013.08.010.
Gill SS, Khan NA, Tuteja N. Differential cadmium stress tolerance in five Indian mustard (Brassica juncea L.) cultivars. Plant Signal Behav. 2011;6:293-300. https://doi.org/10.4161/psb.6.2.15049.
Asati A, Pichhode MNK. Effect of heavy metals on plants: an overview. Int J Appl or Innov Eng Manag. 2016;5:56-66.
Lamers J, van der Meer T, Testerink C. How plants sense and respond to stressful environments. Plant Physiol. 2020;182:1624-35. https://doi.org/10.1104/pp.19.01464.
Devireddy AR, Zandalinas SI, Fichman Y, Mittler R. Integration of reactive oxygen species and hormone signaling during abiotic stress. Plant J. 2021;105:459-76. https://doi.org/10.1111/tpj.15010.
Xia XJ, Zhou YH, Shi K, Zhou J, Foyer CH, Yu JQ. Interplay between reactive oxygen species and hormones in the control of plant development and stress tolerance. J Exp Bot. 2015;66:2839-56. https://doi.org/10.1093/jxb/erv089.
Noctor G, Reichheld JP, Foyer CH. ROS-related redox regulation and signaling in plants. Semin Cell Dev Biol. 2018;80:3-12. https://doi.org/10.1016/j.semcdb.2017.07.013.
Baxter A, Mittler R, Suzuki N. ROS as key players in plant stress signalling. J Exp Bot. 2014;65:1229-40. https://doi.org/10.1093/jxb/ert375.
Nejat N, Mantri N. Plant immune system: Crosstalk between responses to biotic and abiotic stresses the missing link in understanding plant defence. Curr Issues Mol Biol. 2017:1-16. https://doi.org/10.21775/cimb.023.001.
Tsuda K, Katagiri F. Comparing signaling mechanisms engaged in pattern-triggered and effector-triggered immunity. Curr Opin Plant Biol. 2010;13:459-65. https://doi.org/10.1016/j.pbi.2010.04.006.
Schwessinger B, Bart R, Krasileva KV, Coaker G. Focus issue on plant immunity: from model systems to crop species. Front Plant Sci. 2015;6. https://doi.org/10.3389/fpls.2015.00195.
Ding P, Ding Y. Stories of salicylic acid: A plant defense hormone. Trends Plant Sci. 2020;25:549-65. https://doi.org/10.1016/j.tplants.2020.01.004.
Herrera VAisquez A, Salinas P, Holuigue L. Salicylic acid and reactive oxygen species interplay in the transcriptional control of defense genes expression. Front Plant Sci. 2015;6. https://doi.org/10.3389/fpls.2015.00171.
Barra Caracciolo A, Terenzi V. Rhizosphere microbial communities and heavy metals. Microorganisms. 2021;9:1462. https://doi.org/10.3390/microorganisms9071462.
Shakir S, Zaidi SSA, de Vries FT, Mansoor S. Plant genetic networks shaping phyllosphere microbial community. Trends Genet. 2021;37:306-16. https://doi.org/10.1016/j.tig.2020.09.010.
Compant S, Cambon MC, Vacher C, Mitter B, Samad A, Sessitsch A. The plant endosphere world – bacterial life within plants. Environ Microbiol. 2021;23:1812-29. https://doi.org/10.1111/1462-2920.15240.
Trivedi P, Leach JE, Tringe SG, Sa T, Singh BK. Plant–microbiome interactions: from community assembly to plant health. Nat Rev Microbiol. 2020;18:607-21. https://doi.org/10.1038/s41579-020-0412-1.
Kumar V, Jain L, Jain SK, Chaturvedi S, Kaushal P. Bacterial endophytes of rice (Oryza sativa L.) and their potential for plant growth promotion and antagonistic activities. South African J Bot. 2020;134:50-63. https://doi.org/10.1016/j.sajb.2020.02.017.
Mastouri F, Björkman T, Harman GE. Seed treatment with Trichoderma harzianum alleviates biotic, abiotic and physiological stresses in germinating seeds and seedlings. Phytopathology. 2010;100:1213-21. https://doi.org/10.1094/PHYTO-03-10-0091.
Xu Y, Zhang D, Dai L et al. Influence of salt stress on growth of spermosphere bacterial communities in different peanut (Arachis hypogaea L.) cultivars. Int J Mol Sci. 2020;21:2131. https://doi.org/10.3390/ijms21062131.
Basu S, Kumar G, Chhabra S, Prasad R. Role of soil microbes in biogeochemical cycle for enhancing soil fertility. In: Verma JP, Macdonald CA, Gupta VK, Podile AR editor. New and Future Developments in Microbial Biotechnology and Bioengineering. Elsevier. 2021; p. 149-57. https://doi.org/10.1016/B978-0-444-64325-4.00013-4.
Sun H, Jiang S, Jiang C, Wu C, Gao M, Wang Q. A review of root exudates and rhizosphere microbiome for crop production. Environ Sci Pollut Res. 2021;28:54497-510. https://doi.org/10.1007/s11356-021-15838-7.
Wang Y, Ren W, Li Y et al. Nontargeted metabolomic analysis to unravel the impact of di (2-ethylhexyl) phthalate stress on root exudates of alfalfa (Medicago sativa). Sci Total Environ. 2019;646:212-19. https://doi.org/10.1016/j.scitotenv.2018.07.247.
Galloway AF, Knox P, Krause K. Sticky mucilages and exudates of plants: putative microenvironmental design elements with biotechnological value. New Phytol. 2020;225:1461-69. https://doi.org/10.1111/nph.16144.
Zeisler-Diehl VV, Barthlott W, Schreiber L. Plant cuticular waxes: Composition, function and interactions with microorganisms. In: Wilkes H editor. Hydrocarbons, oils and lipids: Diversity, origin, chemistry and fate. Cham: Springer. 2020; p. 123-38. https://doi.org/10.1007/978-3-319-90569-3_7.
Bashir I, War AF, Rafiq I, Reshi ZA, Rashid I, Shouche YS. Phyllosphere microbiome: Diversity and functions. Microbiol Res. 2022;254:126888. https://doi.org/10.1016/j.micres.2021.126888.
Jha P, Panwar J, Jha PN. Mechanistic insights on plant root colonization by bacterial endophytes: a symbiotic relationship for sustainable agriculture. Environ Sustain. 2018;1:25-38. https://doi.org/10.1007/s42398-018-0011-5.
El-Maraghy S, Tohamy A, Hussein K. Plant protection properties of the Plant Growth Promoting Fungi (PGPF): Mechanisms and potentiality. Curr Res Environ Appl Mycol. 2021;11:391-415. https://doi.org/10.5943/cream/11/1/29.
Etesami H, Jeong BR, Glick BR. Contribution of arbuscular mycorrhizal fungi, phosphate–solubilizing bacteria and silicon to P uptake by plant. Front Plant Sci. 2021;12. https://doi.org/10.3389/fpls.2021.699618.
Menka Tiwari, Prashant Singh. Plant defense priming: A new tool for sustainable global food security. In: Shekhawat GS editor. Agricultural Innovations and Sustainability. Agrobios Research. 2021; p. 133-53.
Chakraborty BN, Chakraborty U. Molecular detection of fungal pathogens and induction of phytoimmunity using bioinoculants. Indian Phytopathol. 2021;74:307-22. https://doi.org/10.1007/s42360-021-00351-1.
Zehra A, Raytekar NA, Meena M, Swapnil P. Efficiency of microbial bio-agents as elicitors in plant defense mechanism under biotic stress: A review. Curr Res Microb Sci. 2021;2:100054. https://doi.org/10.1016/j.crmicr.2021.100054.
Mitra D, Djebaili R, Pellegrini M et al. Arbuscular mycorrhizal symbiosis: plant growth improvement and induction of resistance under stressful conditions. J Plant Nutr. 2021;44:1993-2028. https://doi.org/10.1080/01904167.2021.1881552.
Cheng HQ, Giri B, Wu QS, Zou YN, Ku?a K. Arbuscular mycorrhizal fungi mitigate drought stress in citrus by modulating root micro environment. Arch Agron Soil Sci. 2022;68:1217-28. https://doi.org/10.1080/03650340.2021.1878497.
Basyal B, Emery SM. An arbuscular mycorrhizal fungus alters switchgrass growth, root architecture and cell wall chemistry across a soil moisture gradient. Mycorrhiza. 2021;31:251-58. https://doi.org/10.1007/s00572-020-00992-6.
Ruzicka DR, Hausmann NT, Barrios-Masias FH, Jackson LE, Schachtman DP. Transcriptomic and metabolic responses of mycorrhizal roots to nitrogen patches under field conditions. Plant Soil. 2012;350:145-62. https://doi.org/10.1007/s11104-011-0890-z.
Koegel S, Ait Lahmidi N, Arnould C et al. The family of ammonium transporters (AMT) in s orghum bicolor: Two AMT members are induced locally, but not systemically in roots colonized by arbuscular mycorrhizal fungi. New Phytol. 2013;198:853-65. https://doi.org/10.1111/nph.12199.
Zhao Y, Liu Z, Duan F et al. Overexpression of the maize ZmAMT1;1a gene enhances root ammonium uptake efficiency under low ammonium nutrition. Plant Biotechnol Rep. 2018;12:47-56. https://doi.org/10.1007/s11816-018-0471-1.
Wang Y, Zhou W, Wu J, Xie K, Li X. LjAMT2;2 promotes ammonium nitrogen transport during arbuscular mycorrhizal fungi symbiosis in Lotus japonicus. Int J Mol Sci. 2022;23:9522. https://doi.org/10.3390/ijms23179522.
Batra P, Barkodia M, Ahlawat U, Sansanwal R, Sharma T, Wati L. Endophytes: An environmental friendly bacteria for plant growth promotion. Int J Curr Microbiol Appl Sci. 2018;7:1899-911. https://doi.org/10.20546/ijcmas.2018.702.229.
Soni R, Yadav SK, Rajput AS. ACC-deaminase producing rhizobacteria: Prospects and application as stress busters for stressed agriculture. In: Panpatte D, Jhala Y, Shelat H, Vyas R editor. Singapore: Springer. 2018; p. 161-75. https://doi.org/10.1007/978-981-10-7146-1_9.
Ezeh CC, Obi CJ, Moneke AN. Application of microbial synthesized phytohormones in the management of environmental impacts on soils. Bio-Research. 2022;20:1409-25. https://doi.org/10.4314/br.v20i1.3.
Kumar A, Teja ES, Mathur V, Kumari R. Phosphate-solubilizing fungi: Current perspective, mechanisms and potential agricultural applications. In: Yadav A, Mishra S, Kour D, Yadav N, Kumar A editor. Cham: Springer. 2020; p. 121-41. https://doi.org/10.1007/978-3-030-45971-0_6.
Kumar S, Diksha, Sindhu SS, Kumar R. Biofertilizers: An ecofriendly technology for nutrient recycling and environmental sustainability. Curr Res Microb Sci. 2022;3:100094. https://doi.org/10.1016/j.crmicr.2021.100094.
Fukami J, Cerezini P, Hungria M. Azospirillum: benefits that go far beyond biological nitrogen fixation. AMB Express. 2018;8:73. https://doi.org/10.1186/s13568-018-0608-1.
Akbari E, Rasekh B, Maal KB et al. A novel biosurfactant producing Kocuria rosea ABR6 as potential strain in oil sludge recovery and lubrication. AMB Express. 2021;11:131. https://doi.org/10.1186/s13568-021-01283-9.
Thakor R, Mistry H, Bariya H. Isolation and identification of nitrogen fixing bacteria: Azoarcus spp.. In: Amaresan N, Patel P, Amin D editor. Practical handbook on agricultural microbiology. New York: Springer. 2022; p. 47-55. https://doi.org/10.1007/978-1-0716-1724-3_6.
Prakash G, Soni R, Mishra R, Sharma S. Role of plant-microbe interaction in phytoremediation. In: Kumar M, Muthusamy A, Kumar V, Bhalla-Sarin N editor. In vitro plant breeding towards novel agronomic traits. Singapore: Springer. 2019;p.83-118. https://doi.org/10.1007/978-981-32-9824-8_6.
Dhuldhaj U, Pandya U. Diversity, function and application of fungal iron chelators (siderophores) for integrated disease management. In: Seneviratne G, Zavahir JS editor. Role of microbial communities for sustainability. Microorganisms for Sustainability. Singapore: Springer. 2021; p. 259-88. https://doi.org/10.1007/978-981-15-9912-5_10.
Fincheira P, Quiroz A, Tortella G, Diez MC, Rubilar O. Current advances in plant-microbe communication via volatile organic compounds as an innovative strategy to improve plant growth. Microbiol Res. 2021;247:126726. https://doi.org/10.1016/j.micres.2021.126726.
Thomas L, Singh I. Microbe-mediated biotic stress signaling and resistance mechanisms in plants. In: Giri B, Sharma MP editor. Plant Stress Biology. Singapore: Springer. 2020; p. 297-334. https://doi.org/10.1007/978-981-15-9380-2_10.
Yadav B, Jogawat A, Rahman MS, Narayan OP. Secondary metabolites in the drought stress tolerance of crop plants: A review. Gene Reports. 2021;23:101040. https://doi.org/10.1016/j.genrep.2021.101040.
Poveda J, Zabalgogeazcoa I, Soengas P et al. Brassica oleracea var. acephala (Kale) improvement by biological activity of root endophytic fungi. Sci Rep. 2020;10:20224. https://doi.org/10.1038/s41598-020-77215-7.
Phour M, Sehrawat A, Sindhu SS, Glick BR. Interkingdom signaling in plant-rhizomicrobiome interactions for sustainable agriculture. Microbiol Res. 2020;241:126589. https://doi.org/10.1016/j.micres.2020.126589.
Mattoo AJ, Nonzom S. Investigating diverse methods for inducing sporulation in endophytic fungi. Stud Fungi. 2022:1-10. https://doi.org/10.48130/SIF-2022-0016.
Rajpoot R, Srivastava RK, Rani A, Pandey P, Dubey RS. Manganese-induced oxidative stress, ultrastructural changes and proteomics studies in rice plants. Protoplasma. 2021;258:319-35. https://doi.org/10.1007/s00709-020-01575-0.
Sharma M, Sudheer S, Usmani Z, Rani R, Gupta P. Deciphering the omics of plant-microbe interaction: Perspectives and new insights. Curr Genomics. 2020;21:343-62. https://doi.org/10.2174/1389202921999200515140420.
Sandrini M, Nerva L, Sillo F, Balestrini R, Chitarra W, Zampieri E. Abiotic stress and below ground microbiome: The potential of omics approaches. Int J Mol Sci. 2022;23:1091. https://doi.org/10.3390/ijms23031091.
Priya P, Aneesh B, Harikrishnan K. Genomics as a potential tool to unravel the rhizosphere microbiome interactions on plant health. J Microbiol Methods. 2021;185:106215. https://doi.org/10.1016/j.mimet.2021.106215.
Akpinar BA, Kantar M, Budak H. Root precursors of microRNAs in wild emmer and modern wheats show major differences in response to drought stress. Funct Integr Genomics. 2015;15:587-98. https://doi.org/10.1007/s10142-015-0453-0.
Wang Y, Hu B, Du S, Gao S, Chen X, Chen D. Proteomic analyses reveal the mechanism of Dunaliella salina Ds-26-16 gene enhancing salt tolerance in Escherichia coli. Dai S editor. PLoS One. 2016;11:e0153640. https://doi.org/10.1371/journal.pone.0153640.
Khatabi B, Gharechahi J, Ghaffari MR et al. Plant–microbe symbiosis: What has proteomics taught us? Proteomics. 2019;19:1800105. https://doi.org/10.1002/pmic.201800105.
Knief C. Analysis of plant microbe interactions in the era of next generation sequencing technologies. Front Plant Sci. 2014;5. https://doi.org/10.3389/fpls.2014.00216.
Melcher U, Verma R, Schneider WL. Metagenomic search strategies for interactions among plants and multiple microbes. Front Plant Sci. 2014;5. https://doi.org/10.3389/fpls.2014.00268.
Meena KK, Sorty AM, Bitla UM et al. Abiotic stress responses and microbe-mediated mitigation in plants: The omics strategies. Front Plant Sci. 2017;8. https://doi.org/10.3389/fpls.2017.00172.
Hone H, Mann R, Yang G et al. Profiling, isolation and characterisation of beneficial microbes from the seed microbiomes of drought tolerant wheat. Sci Rep. 2021;11:11916. https://doi.org/10.1038/s41598-021-91351-8.
Marcelino VR, Irinyi L, Eden J-S, Meyer W, Holmes EC, Sorrell TC. Metatranscriptomics as a tool to identify fungal species and subspecies in mixed communities – a proof of concept under laboratory conditions. IMA Fungus. 2019;10:12. https://doi.org/10.1186/s43008-019-0012-8.
Bharti MK, Siddique RA, Ranjan K, Chandra D, Singh NP. Relevance of metatranscriptomics in symbiotic associations between plants and rhizosphere microorganisms. In: Nath M, Bhatt D, Bhargava P, Choudhary DK editor. Microbial metatranscriptomics belowground. Singapore: Springer. 2021; p. 59-90. https://doi.org/10.1007/978-981-15-9758-9_3.
Shrestha HK, Appidi MR, Villalobos Solis MI et al. Metaproteomics reveals insights into microbial structure, interactions and dynamic regulation in defined communities as they respond to environmental disturbance. BMC Microbiol. 2021;21:308. https://doi.org/10.1186/s12866-021-02370-4.
Xia Y, Petti C, Williams MA, DeBolt S. Experimental approaches to study plant cell walls during plant-microbe interactions. Front Plant Sci. 2014;5. https://doi.org/10.3389/fpls.2014.00540.
Luan Y, Cui J, Zhai J, Li J, Han L, Meng J. High-throughput sequencing reveals differential expression of miRNAs in tomato inoculated with Phytophthora infestans. Planta. 2015;241:1405-16. https://doi.org/10.1007/s00425-015-2267-7.
Gamalero E, Bona E, Glick BR. Current techniques to study beneficial plant-microbe interactions. Microorganisms. 2022;10:1380. https://doi.org/10.3390/microorganisms10071380.
Munns R, Tester M. Mechanisms of salinity tolerance. Annu Rev Plant Biol. 2008;59:651-81. https://doi.org/10.1146/annurev.arplant.59.032607.092911.
Lew S, Gli?ska-Lewczuk K, Burandt P, Kulesza K, Kobus S, Obolewski K. Salinity as a determinant structuring microbial communities in coastal lakes. Int J Environ Res Public Health. 2022;19:4592. https://doi.org/10.3390/ijerph19084592.
Mishra P, Mishra J, Arora NK. Plant growth promoting bacteria for combating salinity stress in plants – Recent developments and prospects: A review. Microbiol Res. 2021;252:126861. https://doi.org/10.1016/j.micres.2021.126861.
Ilangumaran G, Smith DL. Plant growth promoting rhizobacteria in amelioration of salinity stress: A systems biology perspective. Front Plant Sci. 2017;8. https://doi.org/10.3389/fpls.2017.01768.
Numan M, Bashir S, Khan Y et al. Plant growth promoting bacteria as an alternative strategy for salt tolerance in plants: A review. Microbiol Res. 2018;209:21-32. https://doi.org/10.1016/j.micres.2018.02.003.
Mokrani S, Nabti E, Cruz C. Current advances in plant growth promoting bacteria alleviating salt stress for sustainable agriculture. Appl Sci. 2020;10:7025. https://doi.org/10.3390/app10207025.
Kumawat KC, Nagpal S, Sharma P. Potential of plant growth-promoting rhizobacteria-plant interactions in mitigating salt stress for sustainable agriculture: A review. Pedosphere. 2022;32:223-45. https://doi.org/10.1016/S1002-0160(21)60070-X.
Das S, Nurunnabi TR, Parveen R et al. Isolation and characterization of indole acetic acid producing bacteria from rhizosphere soil and their effect on seed germination. Int J Curr Microbiol Appl Sci. 2019;8:1237-45. https://doi.org/10.20546/ijcmas.2019.803.146.
Zhang BX, Li PS, Wang YY et al. Characterization and synthesis of indole-3-acetic acid in plant growth promoting Enterobacter sp. RSC Adv. 2021;11:31601-607. https://doi.org/10.1039/D1RA05659J.
Kim K, Jang YJ, Lee SM, Oh BT, Chae JC, Lee KJ. Alleviation of salt stress by Enterobacter sp. EJ01 in tomato and Arabidopsis is accompanied by up-regulation of conserved salinity responsive factors in plants. Mol Cells. 2014;37:109-17. https://doi.org/10.14348/molcells.2014.2239.
Kadmiri IM, Chaouqui L, Azaroual SE, Sijilmassi B, Yaakoubi K, Wahby I. Phosphate-solubilizing and auxin-producing rhizobacteria promote plant growth under saline conditions. Arab J Sci Eng. 2018;43:3403-15. https://doi.org/10.1007/s13369-017-3042-9.
Metoui Ben Mahmoud O, Hidri R, Talbi-Zribi O, Taamalli W, Abdelly C, Djébali N. Auxin and proline producing rhizobacteria mitigate salt-induced growth inhibition of barley plants by enhancing water and nutrient status. South African J Bot. 2020;128:209-17. https://doi.org/10.1016/j.sajb.2019.10.023.
Glick BR. Modulation of plant ethylene levels by the bacterial enzyme ACC deaminase. FEMS Microbiol Lett. 2005;251:1-7. https://doi.org/10.1016/j.femsle.2005.07.030.
Arshadullah M, Hyder SI, Mahmood IA, Sultan T NS. Mitigation of salt stress in wheat plant (Triticum aestivum) by plant growth promoting rhizobacteria for ACC deaminase. Int J Adv Res Biol Sci. 2017;4:41-46.
Orozco-Mosqueda M del C, Glick BR, Santoyo G. ACC deaminase in plant growth-promoting bacteria (PGPB): An efficient mechanism to counter salt stress in crops. Microbiol Res. 2020;235:126439. https://doi.org/10.1016/j.micres.2020.126439.
Lee GW, Lee KJ, Chae JC. Herbaspirillum sp. strain GW103 alleviates salt stress in Brassica rapa L. ssp. pekinensis. Protoplasma. 2016;253:655-61. https://doi.org/10.1007/s00709-015-0872-8.
Sarkar A, Ghosh PK, Pramanik K et al. A halotolerant Enterobacter sp. displaying ACC deaminase activity promotes rice seedling growth under salt stress. Res Microbiol. 2018;169:20-32. https://doi.org/10.1016/j.resmic.2017.08.005.
Gupta S, Pandey S. ACC deaminase producing bacteria with multifarious plant growth promoting traits alleviates salinity stress in french bean (Phaseolus vulgaris) plants. Front Microbiol. 2019;10. https://doi.org/10.3389/fmicb.2019.01506.
Kang SM, Shahzad R, Bilal S et al. Indole-3-acetic-acid and ACC deaminase producing Leclercia adecarboxylata MO1 improves Solanum lycopersicum L. growth and salinity stress tolerance by endogenous secondary metabolites regulation. BMC Microbiol. 2019;19:80. https://doi.org/10.1186/s12866-019-1450-6.
Mehmood S, Khan AA, Shi F et al. Alleviation of salt stress in wheat seedlings via multifunctional Bacillus aryabhattai PM34: An in vitro study. Sustainability. 2021;13:8030. https://doi.org/10.3390/su13148030.
Gupta A, Bano A, Rai S et al. ACC deaminase producing plant growth promoting rhizobacteria enhance salinity stress tolerance in Pisum sativum. 3 Biotech. 2021;11:514. https://doi.org/10.1007/s13205-021-03047-5.
Egamberdieva D, Jabborova D, Wirth S. Alleviation of salt stress in legumes by co-inoculation with Pseudomonas and Rhizobium. In: Arora NK editor. Plant Microbe Symbiosis: Fundamentals and Advances. New Delhi: Springer India. 2013; p. 291-303. https://doi.org/10.1007/978-81-322-1287-4_11.
Egamberdieva D, Li L, Lindström K, Räsänen LA. A synergistic interaction between salt-tolerant Pseudomonas and Mesorhizobium strains improves growth and symbiotic performance of liquorice (Glycyrrhiza uralensis Fish.) under salt stress. Appl Microbiol Biotechnol. 2016;100:2829-41. https://doi.org/10.1007/s00253-015-7147-3.
Abdiev A, Khaitov B, Toderich K, Park KW. Growth, nutrient uptake and yield parameters of chickpea (Cicer arietinum L.) enhance by Rhizobium and Azotobacter inoculations in saline soil. J Plant Nutr. 2019;42:2703-14. https://doi.org/10.1080/01904167.2019.1655038.
Irshad A, Rehman RNU, Abrar MM, Saeed Q, Sharif R, Hu T. Contribution of rhizobium–legume symbiosis in salt stress tolerance in Medicago truncatula evaluated through photosynthesis, antioxidant enzymes and compatible solutes accumulation. Sustainability. 2021;13:3369. https://doi.org/10.3390/su13063369.
Ilangumaran G, Schwinghamer TD, Smith DL. Rhizobacteria from root nodules of an indigenous legume enhance salinity stress tolerance in soybean. Front Sustain Food Syst. 2021;4. https://doi.org/10.3389/fsufs.2020.617978.
Metwali1 EMR, Abdelmoneim TS, Bakheit MA, Kadasa NMS. Alleviation of salinity stress in faba bean (Vicia faba L.) plants by inoculation with plant growth promoting rhizobacteria (PGPR). POJ. 2015;8:449-60.
Raheem A, Ali B. Halotolerant rhizobacteria: beneficial plant metabolites and growth enhancement of Triticum aestivum L. in salt-amended soils. Arch Agron Soil Sci. 2015;61:1691-705. https://doi.org/10.1080/03650340.2015.1036044.
Kasim WA, Gaafar RM, Abou-Ali RM, Omar MN, Hewait HM. Effect of biofilm forming plant growth promoting rhizobacteria on salinity tolerance in barley. Ann Agric Sci. 2016;61:217-27. https://doi.org/10.1016/j.aoas.2016.07.003.
Hahm MS, Son JS, Hwang YJ, Kwon DK, Ghim SY. Alleviation of salt stress in pepper (Capsicum annum L.) plants by plant growth-promoting rhizobacteria. J Microbiol Biotechnol. 2017;27:1790-97. https://doi.org/10.4014/jmb.1609.09042.
Yasin NA, Khan WU, Ahmad SR, Ali A, Ahmad A, Akram W. Imperative roles of halotolerant plant growth-promoting rhizobacteria and kinetin in improving salt tolerance and growth of black gram (Phaseolus mungo). Environ Sci Pollut Res. 2018;25:4491-505. https://doi.org/10.1007/s11356-017-0761-0.
Ansari M, Shekari F, Mohammadi MH, Juhos K, Végvári G, Biró B. Salt-tolerant plant growth-promoting bacteria enhanced salinity tolerance of salt-tolerant alfalfa (Medicago sativa L.) cultivars at high salinity. Acta Physiol Plant. 2019;41:195. https://doi.org/10.1007/s11738-019-2988-5.
Kearl J, McNary C, Lowman JS et al. Salt-tolerant halophyte rhizosphere bacteria stimulate growth of alfalfa in salty soil. Front Microbiol. 2019;10. https://doi.org/10.3389/fmicb.2019.01849.
Chu TN, Tran BTH, Van Bui L, Hoang MTT. Plant growth-promoting rhizobacterium Pseudomonas PS01 induces salt tolerance in Arabidopsis thaliana. BMC Res Notes. 2019;12:11. https://doi.org/10.1186/s13104-019-4046-1.
Shultana R, Kee Zuan AT, Yusop MR, Saud HM. Characterization of salt-tolerant plant growth-promoting rhizobacteria and the effect on growth and yield of saline-affected rice. Lopes AR, editor. PLoS One. 2020;15:e0238537. https://doi.org/10.1371/journal.pone.0238537.
Yasmeen T, Ahmad A, Arif MS et al. Biofilm forming rhizobacteria enhance growth and salt tolerance in sunflower plants by stimulating antioxidant enzymes activity. Plant Physiol Biochem. 2020;156:242-56. https://doi.org/10.1016/j.plaphy.2020.09.016.
Nawaz A, Shahbaz M, Asadullah et al. Potential of salt tolerant PGPR in growth and yield augmentation of wheat (Triticum aestivum L.) under saline conditions. Front Microbiol. 2020;11. https://doi.org/10.3389/fmicb.2020.02019.
Wang R, Wang C, Feng Q, Liou RM, Lin YF. Biological inoculant of salt-tolerant bacteria for plant growth stimulation under different saline soil conditions. J Microbiol Biotechnol. 2021;31:398-407. https://doi.org/10.4014/jmb.2009.09032.
Sapre S, Gontia-Mishra I, Tiwari S. Plant growth-promoting rhizobacteria ameliorates salinity stress in pea (Pisum sativum). J Plant Growth Regul. 2022;41:647-56. https://doi.org/10.1007/s00344-021-10329-y.
Baltruschat H, Fodor J, Harrach BD et al. Salt tolerance of barley induced by the root endophyte Piriformospora indica is associated with a strong increase in antioxidants. New Phytol. 2008;180:501-10. https://doi.org/10.1111/j.1469-8137.2008.02583.x.
Abdelaziz ME, Kim D, Ali S, Fedoroff NV, Al-Babili S. The endophytic fungus Piriformospora indica enhances Arabidopsis thaliana growth and modulates Na + /K + homeostasis under salt stress conditions. Plant Sci. 2017;263:107-15. https://doi.org/10.1016/j.plantsci.2017.07.006.
Sharma P, Kharkwal AC, Abdin MZ, Varma A. Piriformospora indica-mediated salinity tolerance in Aloe vera plantlets. Symbiosis. 2017;72:103-15. https://doi.org/10.1007/s13199-016-0449-0.
Ghorbani A, Omran VOG, Razavi SM, Pirdashti H, Ranjbar M. Piriformospora indica confers salinity tolerance on tomato (Lycopersicon esculentum Mill.) through amelioration of nutrient accumulation, K+/Na+ homeostasis and water status. Plant Cell Rep. 2019;38:1151-163. https://doi.org/10.1007/s00299-019-02434-w.
Sampangi-Ramaiah MH, Jagadheesh, Dey P et al. An endophyte from salt-adapted Pokkali rice confers salt-tolerance to a salt-sensitive rice variety and targets a unique pattern of genes in its new host. Sci Rep. 2020;10:3237. https://doi.org/10.1038/s41598-020-59998-x.
Sepehri M, Ghaffari MR, Khayam Nekoui M et al. Root endophytic fungus Serendipita indica modulates barley leaf blade proteome by increasing the abundance of photosynthetic proteins in response to salinity. J Appl Microbiol. 2021;131:1870-89. https://doi.org/10.1111/jam.15063.
Gamalero E, Berta G, Glick BR. The use of microorganisms to facilitate the growth of plants in saline soils. In: Khan M, Zaidi A, Musarrat J editor. Microbial strategies for crop improvement. Berlin, Heidelberg: Springer. 2009; p. 1-22. https://doi.org/10.1007/978-3-642-01979-1_1.
Iqbal N, Umar S, Khan NA, Khan MIR. A new perspective of phytohormones in salinity tolerance: Regulation of proline metabolism. Environ Exp Bot. 2014;100:34-42. https://doi.org/10.1016/j.envexpbot.2013.12.006.
Abeer H, Abd_Allah EF, Alqarawi AA, Egamberdieva D. Induction of salt stress tolerance in cowpea [Vigna unguiculata (L.) Walp.] by arbuscular mycorrhizal fungi. Legum Res - An Int J. 2015;38. https://doi.org/https://doi.org/10.18805/lr.v38i5.5933.
Hashem A, Abd_Allah EF, Alqarawi AA, Wirth S, Egamberdieva D. Arbuscular mycorrhizal fungi alleviate salt stress in lupine (Lupinus termis Forsik) through modulation of antioxidant defense systems and physiological traits. Legum Res - An Int J. 2016;39. https://doi.org/https://doi.org/10.18805/lr.v39i2.9531.
Hashem A, Abd_Allah EF, Alqarawi AA, Aldubise A, Egamberdieva D. Arbuscular mycorrhizal fungi enhances salinity tolerance of Panicum turgidum Forssk by altering photosynthetic and antioxidant pathways. J Plant Interact. 2015;10:230-42. https://doi.org/10.1080/17429145.2015.1052025.
Elhindi KM, El-Din AS, Elgorban AM. The impact of arbuscular mycorrhizal fungi in mitigating salt-induced adverse effects in sweet basil (Ocimum basilicum L.). Saudi J Biol Sci. 2017;24:170-79. https://doi.org/10.1016/j.sjbs.2016.02.010.
Shi-chu L, Yong J, Ma-bo L, Wen-xu Z, Nan X, Hui-hui Z. Improving plant growth and alleviating photosynthetic inhibition from salt stress using AMF in alfalfa seedlings. J Plant Interact. 2019;14:482-91. https://doi.org/10.1080/17429145.2019.1662101.
Wang, Sun, Shi. Arbuscular mycorrhiza enhances biomass production and salt tolerance of sweet sorghum. Microorganisms. 2019;7:289. https://doi.org/10.3390/microorganisms7090289.
Parvin S, Van Geel M, Yeasmin T, Verbruggen E, Honnay O. Effects of single and multiple species inocula of arbuscular mycorrhizal fungi on the salinity tolerance of a Bangladeshi rice (Oryza sativa L.) cultivar. Mycorrhiza. 2020;30:431-44. https://doi.org/10.1007/s00572-020-00957-9.
Klinsukon C, Lumyong S, Kuyper TW, Boonlue S. Colonization by arbuscular mycorrhizal fungi improves salinity tolerance of eucalyptus (Eucalyptus camaldulensis) seedlings. Sci Rep. 2021;11:4362. https://doi.org/10.1038/s41598-021-84002-5.
Wang J, Yuan J, Ren Q et al. Arbuscular mycorrhizal fungi enhanced salt tolerance of Gleditsia sinensis by modulating antioxidant activity, ion balance and P/N ratio. Plant Growth Regul. 2022;97:33-49. https://doi.org/10.1007/s10725-021-00792-8.
Nisha Kadian KY, AA. Application of AM Fungi with Bradyrhizobium japonicum in improving growth, nutrient uptake and yield of Vigna radiata L. under saline soil. J Stress Physiol Biochem. 2014;10:134-52.
Krishnamoorthy R, Kim K, Subramanian P, Senthilkumar M, Anandham R, Sa T. Arbuscular mycorrhizal fungi and associated bacteria isolated from salt-affected soil enhances the tolerance of maize to salinity in coastal reclamation soil. Agric Ecosyst Environ. 2016;231:233-39. https://doi.org/10.1016/j.agee.2016.05.037.
Abdel Motaleb NA, Abd Elhady SA, Ghoname AA. AMF and Bacillus megaterium neutralize the harmful effects of salt stress on bean plants. Gesunde Pflanz. 2020;72:29-39. https://doi.org/10.1007/s10343-019-00480-8.
Hanaka A, Ozimek E, Reszczy?ska E, Jaroszuk-?cise? J, Stolarz M. Plant tolerance to drought stress in the presence of supporting bacteria and fungi: An efficient strategy in horticulture. Horticulturae. 2021;7:390. https://doi.org/10.3390/horticulturae7100390.
Abdelaal K, AlKahtani M, Attia K, Hafez Y, Király L, Künstler A. The role of plant growth-promoting bacteria in alleviating the adverse effects of drought on plants. Biology (Basel). 2021;10:520. https://doi.org/10.3390/biology10060520.
Bogati K, Walczak M. The impact of drought stress on soil microbial community, enzyme activities and plants. Agronomy. 2022;12:189. https://doi.org/10.3390/agronomy12010189.
Shrivastava P, Kumar R. Soil salinity: A serious environmental issue and plant growth promoting bacteria as one of the tools for its alleviation. Saudi J Biol Sci. 2015;22:123-31. https://doi.org/10.1016/j.sjbs.2014.12.001.
Marasco R, Rolli E, Vigani G et al. Are drought-resistance promoting bacteria cross-compatible with different plant models? Plant Signal Behav. 2013;8:e26741. https://doi.org/10.4161/psb.26741.
Niu X, Song L, Xiao Y, Ge W. Drought-tolerant plant growth-promoting rhizobacteria associated with foxtail millet in a semi-arid agroecosystem and their potential in alleviating drought stress. Front Microbiol. 2018;8. https://doi.org/10.3389/fmicb.2017.02580.
Saikia J, Sarma RK, Dhandia R et al. Alleviation of drought stress in pulse crops with ACC deaminase producing rhizobacteria isolated from acidic soil of Northeast India. Sci Rep. 2018;8:3560. https://doi.org/10.1038/s41598-018-21921-w.
Liu J, Qiu G, Liu C et al. Salicylic acid, a multifaceted hormone, combats abiotic stresses in plants. Life. 2022;12:886. https://doi.org/10.3390/life12060886.
Lastochkina O, Ivanov S, Petrova S et al. Role of endogenous salicylic acid as a hormonal intermediate in the bacterial endophyte Bacillus subtilis-induced protection of wheat genotypes contrasting in drought susceptibility under dehydration. Plants. 2022;11:3365. https://doi.org/10.3390/plants11233365.
Milosevic N, Marinkovic J, Tintor B. Mitigating abiotic stress in crop plants by microorganisms. Zb Matice Srp za Prir Nauk. 2012;17-26. https://doi.org/https://doi.org/10.2298/ZMSPN1223017M.
Sayer EJ, Crawford JA, Edgerley J et al. Adaptation to chronic drought modifies soil microbial community responses to phytohormones. Commun Biol. 2021;4:516. https://doi.org/10.1038/s42003-021-02037-w.
Wu QS, He JD, Srivastava AK, Zou YN, Ku?a K. Mycorrhizas enhance drought tolerance of citrus by altering root fatty acid compositions and their saturation levels. Polle A editor. Tree Physiol. 2019;39:1149-58. https://doi.org/10.1093/treephys/tpz039.
He JD, Zou YN, Wu QS, Ku?a K. Mycorrhizas enhance drought tolerance of trifoliate orange by enhancing activities and gene expression of antioxidant enzymes. Sci Hortic (Amsterdam). 2020;262:108745. https://doi.org/10.1016/j.scienta.2019.108745.
Zou YN, Wu QS, Ku?a K. Unravelling the role of arbuscular mycorrhizal fungi in mitigating the oxidative burst of plants under drought stress. Hu Y editor. Plant Biol. 2021;23:50-57. https://doi.org/10.1111/plb.13161.
Wu HH, Zou YN, Rahman MM, Ni QD, Wu QS. Mycorrhizas alter sucrose and proline metabolism in trifoliate orange exposed to drought stress. Sci Rep. 2017;7:42389. https://doi.org/10.1038/srep42389.
Pavithra D, Yapa N. Arbuscular mycorrhizal fungi inoculation enhances drought stress tolerance of plants. Groundw Sustain Dev. 2018;7:490-94. https://doi.org/10.1016/j.gsd.2018.03.005.
Hashem A, Kumar A, Al-Dbass AM et al. Arbuscular mycorrhizal fungi and biochar improves drought tolerance in chickpea. Saudi J Biol Sci. 2019;26:614-24. https://doi.org/10.1016/j.sjbs.2018.11.005.
Moradtalab N, Hajiboland R, Aliasgharzad N, Hartmann TE, Neumann G. Silicon and the association with an arbuscular-mycorrhizal fungus (Rhizophagus clarus) mitigate the adverse effects of drought stress on strawberry. Agronomy. 2019;9:41. https://doi.org/10.3390/agronomy9010041.
Mathur S, Tomar RS, Jajoo A. Arbuscular mycorrhizal fungi (AMF) protects photosynthetic apparatus of wheat under drought stress. Photosynth Res. 2019;139:227-38. https://doi.org/10.1007/s11120-018-0538-4.
Li J, Meng B, Chai H et al. Arbuscular mycorrhizal fungi alleviate drought stress in C3 (Leymus chinensis) and C4 (Hemarthria altissima) grasses via altering antioxidant enzyme activities and photosynthesis. Front Plant Sci. 2019;10. https://doi.org/10.3389/fpls.2019.00499.
Cheng HQ, Zou YN, Wu QS, Ku?a K. Arbuscular mycorrhizal fungi alleviate drought stress in trifoliate orange by regulating H+-ATPase activity and gene expression. Front Plant Sci. 2021;12. https://doi.org/10.3389/fpls.2021.659694.
Gholinezhad E, Darvishzadeh R. Influence of arbuscular mycorrhiza fungi and drought stress on fatty acids profile of sesame (Sesamum indicum L.). F Crop Res. 2021;262:108035. https://doi.org/10.1016/j.fcr.2020.108035.
Behrooz A, Vahdati K, Rejali F, Lotfi M, Sarikhani S, Leslie C. Arbuscular mycorrhiza and plant growth-promoting bacteria alleviate drought stress in walnut. HortScience. 2019;54:1087-92. https://doi.org/10.21273/HORTSCI13961-19.
Arun K. D, Sabarinathan KG, Gomathy M, Kannan R, Balachandar D. Mitigation of drought stress in rice crop with plant growth-promoting abiotic stress-tolerant rice phyllosphere bacteria. J Basic Microbiol. July 2020. https://doi.org/10.1002/jobm.202000011.
Sharath S, Triveni S, Nagaraju Y, Latha PC, Vidyasagar B. The role of phyllosphere bacteria in improving cotton growth and yield under drought conditions. Front Agron. 2021;3. https://doi.org/10.3389/fagro.2021.680466.
Paradiso R, Arena C, De Micco V, Giordano M, Aronne G, De Pascale S. Changes in leaf anatomical traits enhanced photosynthetic activity of soybean grown in hydroponics with plant growth-promoting microorganisms. Front Plant Sci. 2017;8. https://doi.org/10.3389/fpls.2017.00674.
Mo Y, Wang Y, Yang R et al. Regulation of plant growth, photosynthesis, antioxidation and osmosis by an arbuscular mycorrhizal fungus in watermelon seedlings under well-watered and drought conditions. Front Plant Sci. 2016;7. https://doi.org/10.3389/fpls.2016.00644.
Pal A, Pandey S. Role of arbuscular mycorrhizal fungi on plant growth and reclamation of barren soil with wheat (Triticum aestivum L.) crop. Int J Soil Sci. 2016;12:25-31. https://doi.org/10.3923/ijss.2017.25.31.
Zhang F, He JD, Ni QD, Wu QS, Zou YN. Enhancement of drought tolerance in trifoliate orange by mycorrhiza: Changes in root sucrose and proline metabolisms. Not Bot Horti Agrobot Cluj-Napoca. 2018;46:270-76. https://doi.org/10.15835/nbha46110983.
Ren AT, Zhu Y, Chen YL et al. Arbuscular mycorrhizal fungus alters root-sourced signal (abscisic acid) for better drought acclimation in Zea mays L. seedlings. Environ Exp Bot. 2019;167:103824. https://doi.org/10.1016/j.envexpbot.2019.103824.
Zhang F, Zou YN, Wu QS, Ku?a K. Arbuscular mycorrhizas modulate root polyamine metabolism to enhance drought tolerance of trifoliate orange. Environ Exp Bot. 2020;171:103926. https://doi.org/10.1016/j.envexpbot.2019.103926.
Li F, Duan T, Li Y. Effects of the fungal endophyte Epichloë festucae var. lolii on growth and physiological responses of perennial ryegrass cv. fairway to combined drought and pathogen stresses. Microorganisms. 2020;8:1917. https://doi.org/10.3390/microorganisms8121917.
Li X, He C, He X et al. Dark septate endophytes improve the growth of host and non-host plants under drought stress through altered root development. Plant Soil. 2019;439:259-72. https://doi.org/10.1007/s11104-019-04057-2.
Guler NS, Pehlivan N, Karaoglu SA, Guzel S, Bozdeveci A. Trichoderma atroviride ID20G inoculation ameliorates drought stress-induced damages by improving antioxidant defence in maize seedlings. Acta Physiol Plant. 2016;38:132. https://doi.org/10.1007/s11738-016-2153-3.
Morsy M, Cleckler B, Armuelles-Millican H. Fungal endophytes promote tomato growth and enhance drought and salt tolerance. Plants. 2020;9:877. https://doi.org/10.3390/plants9070877.
Gururani MA, Upadhyaya CP, Baskar V, Venkatesh J, Nookaraju A, Park SW. Plant growth-promoting rhizobacteria enhance abiotic stress tolerance in Solanum tuberosum through inducing changes in the expression of ROS-scavenging enzymes and improved photosynthetic performance. J Plant Growth Regul. 2013;32:245-58. https://doi.org/10.1007/s00344-012-9292-6.
Armada E, Roldán A, Azcon R. Differential activity of autochthonous bacteria in controlling drought stress in native Lavandula and Salvia plants species under drought conditions in natural arid soil. Microb Ecol. 2014;67:410-20. https://doi.org/https://doi.org/10.1007/s00248-013-0326-9.
Barnawal D, Bharti N, Pandey SS, Pandey A, Chanotiya CS, Kalra A. Plant growth-promoting rhizobacteria enhance wheat salt and drought stress tolerance by altering endogenous phytohormone levels and TaCTR1 / TaDREB2 expression. Physiol Plant. 2017;161:502-14. https://doi.org/https://doi.org/10.1111/ppl.12614.
García JE, Maroniche G, Creus C, Suárez-Rodríguez R, Ramirez-Trujillo JA, Groppa MD. In vitro PGPR properties and osmotic tolerance of different Azospirillum native strains and their effects on growth of maize under drought stress. Microbiol Res. 2017;202:21-29. https://doi.org/https://doi.org/10.1016/j.micres.2017.04.007.
Djanaguiraman M, Boyle DL, Welti R, Jagadish SVK, Prasad PVV. Decreased photosynthetic rate under high temperature in wheat is due to lipid desaturation, oxidation, acylation and damage of organelles. BMC Plant Biol. 2018;18:55. https://doi.org/https://doi.org/10.1186/s12870-018-1263-z.
Jia Y, Zou D, Wang J et al. Effects of ?-aminobutyric acid, glutamic acid and calcium chloride on rice (Oryza sativa L.) under cold stress during the early vegetative stage. J Plant Growth Regul. 2017;36:240-53. https://doi.org/https://doi.org/10.1007/s00344-016-9634-x.
Peñuelas J, Rico L, Ogaya R, Jump AS, Terradas J. Summer season and long-term drought increase the richness of bacteria and fungi in the foliar phyllosphere of Quercus ilex in a mixed Mediterranean forest. Plant Biol. 2012;14:565-75. https://doi.org/10.1111/j.1438-8677.2011.00532.x.
Aydogan EL, Moser G, Müller C, Kämpfer P, Glaeser SP. Long-term warming shifts the composition of bacterial communities in the phyllosphere of Galium album in a permanent grassland field-experiment. Front Microbiol. 2018;9. https://doi.org/10.3389/fmicb.2018.00144.
Milosevic N, Marinkovic J, Tintor B. Mitigating abiotic stress in crop plants by microorganisms. Zb Matice Srp za Prir Nauk. 2012:17-26. https://doi.org/10.2298/ZMSPN1223017M.
Abd El-Daim IA, Bejai S, Meijer J. Improved heat stress tolerance of wheat seedlings by bacterial seed treatment. Plant Soil. 2014;379:337-50. https://doi.org/https://doi.org/10.1007/s11104-014-2063-3.
Shin DJ, Yoo SJ, Hong JK, Weon HY, Song J, Sang MK. Effect of Bacillus aryabhattai H26-2 and B. siamensis H30-3 on growth promotion and alleviation of heat and drought stresses in Chinese cabbage. Plant Pathol J. 2019;35:178-87. https://doi.org/https://doi.org/10.5423/PPJ.NT.08.2018.0159.
Khan MA, Asaf S, Khan AL et al. Thermotolerance effect of plant growth-promoting Bacillus cereus SA1 on soybean during heat stress. BMC Microbiol. 2020;20:175. https://doi.org/http//doi.org/10.1186/s12866-020-01822-77.
McLellan CA, Turbyville TJ, Wijeratne EMK et al. A rhizosphere fungus enhances Arabidopsis thermotolerance through production of an HSP90 inhibitor. Plant Physiol. 2007;145:174-82. https://doi.org/10.1104/pp.107.101808.
Waqas M, Khan AL, Shahzad R, Ullah I, Khan AR, Lee IJ. Mutualistic fungal endophytes produce phytohormones and organic acids that promote japonica rice plant growth under prolonged heat stress. J Zhejiang Univ B. 2015;16:1011-18. https://doi.org/10.1631/jzus.B1500081.
Wu QS. Mycorrhizal efficacy of trifoliate orange seedlings on alleviating temperature stress. Plant, Soil Environ. 2011;57:459-64. https://doi.org/10.17221/59/2011-PSE.
Mathur S, Sharma MP, Jajoo A. Improved photosynthetic efficacy of maize (Zea mays) plants with arbuscular mycorrhizal fungi (AMF) under high temperature stress. J Photochem Photobiol B Biol. 2018;180:149-54. https://doi.org/10.1016/j.jphotobiol.2018.02.002.
Cabral C, Ravnskov S, Tringovska I, Wollenweber B. Arbuscular mycorrhizal fungi modify nutrient allocation and composition in wheat (Triticum aestivum L.) subjected to heat-stress. Plant Soil. 2016;408:385-99. https://doi.org/10.1007/s11104-016-2942-x.
Ma J, Sun C, Bai L et al. Transcriptome analysis of cucumber roots reveals key cold-resistance genes induced by AM fungi. Plant Mol Biol Report. 2018;36:135-48. https://doi.org/10.1007/s11105-018-1066-2.
Pasbani B, Salimi A, Aliasgharzad N, Hajiboland R. Colonization with arbuscular mycorrhizal fungi mitigates cold stress through improvement of antioxidant defense and accumulation of protecting molecules in eggplants. Sci Hortic (Amsterdam). 2020;272:109575. https://doi.org/10.1016/j.scienta.2020.109575.
Dastogeer KMG, Zahan MI, Rhaman MS, Sarker MSA, Chakraborty A. Microbe-mediated thermotolerance in plants and pertinent mechanisms- A meta-analysis and review. Front Microbiol. 2022;13. https://doi.org/10.3389/fmicb.2022.833566.
Zhu XC, Song FB, Liu SQ, Liu TD. Effects of arbuscular mycorrhizal fungus on photosynthesis and water status of maize under high temperature stress. Plant Soil. 2011;346:189-99. https://doi.org/10.1007/s11104-011-0809-8.
Abdel Latef AAH, Chaoxing H. Arbuscular mycorrhizal influence on growth, photosynthetic pigments, osmotic adjustment and oxidative stress in tomato plants subjected to low temperature stress. Acta Physiol Plant. 2011;33:1217-25. https://doi.org/10.1007/s11738-010-0650-3.
Chen S, Jin W, Liu A et al. Arbuscular mycorrhizal fungi (AMF) increase growth and secondary metabolism in cucumber subjected to low temperature stress. Sci Hortic (Amsterdam). 2013;160:222-29. https://doi.org/10.1016/j.scienta.2013.05.039.
Maya MA, Matsubara Y. Influence of arbuscular mycorrhiza on the growth and antioxidative activity in cyclamen under heat stress. Mycorrhiza. 2013;23:381-90. https://doi.org/10.1007/s00572-013-0477-z.
Ali SZ, Sandhya V, Grover M, Linga VR, Bandi V. Effect of inoculation with a thermotolerant plant growth promoting Pseudomonas putida strain AKMP7 on growth of wheat (Triticum spp.) under heat stress. J Plant Interact. 2011;6:239-46. https://doi.org/10.1080/17429145.2010.545147.
Issa A, Esmaeel Q, Sanchez L et al. Impacts of Paraburkholderia phytofirmans strain PsJN on tomato (Lycopersicon esculentum L.) under high temperature. Front Plant Sci. 2018;9. https://doi.org/10.3389/fpls.2018.01397.
Bruno LB, Karthik C, Ma Y, Kadirvelu K, Freitas H, Rajkumar M. Amelioration of chromium and heat stresses in Sorghum bicolor by Cr6+ reducing-thermotolerant plant growth promoting bacteria. Chemosphere. 2020;244:125521. https://doi.org/10.1016/j.chemosphere.2019.125521.
Hubbard M, Germida JJ, Vujanovic V. Fungal endophytes enhance wheat heat and drought tolerance in terms of grain yield and second-generation seed viability. J Appl Microbiol. 2014;116:109-22. https://doi.org/10.1111/jam.12311.
Sangamesh MB, Jambagi S, Vasanthakumari MM et al. Thermotolerance of fungal endophytes isolated from plants adapted to the Thar Desert, India. Symbiosis. 2018;75:135-47. https://doi.org/10.1007/s13199-017-0527-y.
Ismail I, Hamayun M, Hussain A, Khan SA, Iqbal ALI. An endophytic fungus Aspergillus violaceofuscus can be used as heat stress adaptive tool for Glycine max L. and Helianthus annuus L. J Appl Bot Food Qual. 2020;93:112-25.
Li X, Zhao C, Zhang T et al. Exogenous Aspergillus aculeatus enhances drought and heat tolerance of perennial Ryegrass. Front Microbiol. 2021;12. https://doi.org/10.3389/fmicb.2021.593722.
Ju W, Liu L, Fang L, Cui Y, Duan C, Wu H. Impact of co-inoculation with plant-growth-promoting rhizobacteria and rhizobium on the biochemical responses of alfalfa-soil system in copper contaminated soil. Ecotoxicol Environ Saf. 2019;167:218-26. https://doi.org/10.1016/j.ecoenv.2018.10.016.
Etesami H, Noori F, Ebadi A, Reiahi Samani N. Alleviation of stress-induced ethylene-mediated negative impact on crop plants by bacterial ACC deaminase: Perspectives and applications in stressed agriculture management. In: Yadav A, Singh J, Rastegari A, Yadav N editor. Plant microbiomes for sustainable agriculture. Sustainable Development and Biodiversity. Cham: Springer. 2020; p. 287-315. https://doi.org/10.1007/978-3-030-38453-1_10.
Ndeddy Aka RJ, Babalola OO. Effect of bacterial inoculation of strains of Pseudomonas aeruginosa, Alcaligenes feacalis and Bacillus subtilis on germination, growth and heavy metal (Cd, Cr and Ni) uptake of Brassica juncea. Int J Phytoremediation. 2016;18:200-09. https://doi.org/10.1080/15226514.2015.1073671.
Pepi M, Borra M, Tamburrino S et al. A Bacillus sp. isolated from sediments of the Sarno River mouth, Gulf of Naples (Italy) produces a biofilm biosorbing Pb(II). Sci Total Environ. 2016;562:588-95. https://doi.org/10.1016/j.scitotenv.2016.04.097.
Suhadolnik MLS, Costa PS, Castro GM, Lobo FP, Nascimento AMA. Comprehensive insights into arsenic- and iron-redox genes, their taxonomy and associated environmental drivers deciphered by a meta-analysis. Environ Int. 2021;146:106234. https://doi.org/10.1016/j.envint.2020.106234.
Sharma RK, Barot K, Archana G. Root colonization by heavy metal resistant Enterobacter and its influence on metal induced oxidative stress on Cajanus cajan. J Sci Food Agric. 2020;100:1532-40. https://doi.org/10.1002/jsfa.10161.
Sharma B, Shukla P. A comparative analysis of heavy metal bioaccumulation and functional gene annotation towards multiple metal resistant potential by Ochrobactrum intermedium BPS-20 and Ochrobactrum ciceri BPS-26. Bioresour Technol. 2021;320:124330. https://doi.org/10.1016/j.biortech.2020.124330.
Li D, Li R, Ding Z et al. Discovery of a novel native Bacterium of Providencia sp. with high biosorption and oxidation ability of manganese for bioleaching of heavy metal contaminated soils. Chemosphere. 2020;241:125039. https://doi.org/10.1016/j.chemosphere.2019.125039.
Bhandari G, Bhatt P. Concepts and application of plant–microbe interaction in remediation of heavy metals. In: Sharma A editor. Microbes and signaling biomolecules against plant stress. Singapore: Springer. 2021; p. 55-77. https://doi.org/10.1007/978-981-15-7094-0_4.
Ashraf MA, Hussain I, Rasheed R, Iqbal M, Riaz M, Arif MS. Advances in microbe-assisted reclamation of heavy metal contaminated soils over the last decade: A review. J Environ Manage. 2017;198:132-43. https://doi.org/10.1016/j.jenvman.2017.04.060.
Sarwar S, Khaliq A, Yousra M, Sultan T, Ahmad N, Khan MZ. Screening of siderophore-producing PGPRs isolated from groundnut (Arachis hypogaea L.) rhizosphere and their influence on iron release in soil. Commun Soil Sci Plant Anal. 2020;51:1680-92. https://doi.org/10.1080/00103624.2020.1791159.
Junpradit C, Thooppeng P, Duangmal K, Prapagdee B. Influence of cadmium-resistant streptomycetes on plant growth and cadmium uptake by Chlorophytum comosum (Thunb.) Jacques. Environ Sci Pollut Res. 2021;28:39398-408. https://doi.org/10.1007/s11356-021-13527-z.
Pathom-aree W, Matako A, Rangseekaew P, Seesuriyachan P, Srinuanpan S. Performance of Actinobacteria isolated from rhizosphere soils on plant growth promotion under cadmium toxicity. Int J Phytoremediation. 2021;23:1497-505. https://doi.org/10.1080/15226514.2021.1913992.
Wang Y, Huang W, Li Y, Yu F, Penttinen P. Isolation, characterization and evaluation of a high-siderophore-yielding bacterium from heavy metal–contaminated soil. Environ Sci Pollut Res. 2022;29:3888-99. https://doi.org/10.1007/s11356-021-15996-8.
Kumar A, Tripti, Maleva M, Bruno LB, Rajkumar M. Synergistic effect of ACC deaminase producing Pseudomonas sp. TR15a and siderophore producing Bacillus aerophilus TR15c for enhanced growth and copper accumulation in Helianthus annuus L. Chemosphere. 2021;276:130038. https://doi.org/10.1016/j.chemosphere.2021.130038.
Chen J, Li N, Han S et al. Characterization and bioremediation potential of nickel-resistant endophytic bacteria isolated from the wetland plant Tamarix chinensis. FEMS Microbiol Lett. 2020;367. https://doi.org/10.1093/femsle/fnaa098.
Kumar S, Saxena S. Arbuscular mycorrhizal fungi (AMF) from heavy metal-contaminated soils: Molecular approach and application in phytoremediation. In: Giri B, Prasad R, Wu QS, Varma A editor. Biofertilizers for sustainable agriculture and environment. Cham: Springer. 2019; p. 489-500. https://doi.org/10.1007/978-3-030-18933-4_22.
Wang G, Wang L, Ma F, You Y, Wang Y, Yang D. Integration of earthworms and arbuscular mycorrhizal fungi into phytoremediation of cadmium-contaminated soil by Solanum nigrum L. J Hazard Mater. 2020;389:121873. https://doi.org/10.1016/j.jhazmat.2019.121873.
Yadav RC, Sharma SK, Ramesh A, Sharma K, Sharma PK, Varma A. Contribution of zinc-solubilizing and -mobilizing microorganisms (ZSMM) to enhance zinc bioavailability for better soil, plant and human health. In: Sharma SK, Singh UB, Sahu PK, Singh HV, Sharma PK editor. Rhizosphere microbes. Microorganisms for Sustainability. Singapore: Springer. 2020; p. 357-86. https://doi.org/10.1007/978-981-15-9154-9_14.
Malik A, Butt TA, Naqvi STA et al. Lead tolerant endophyte Trametes hirsuta improved the growth and lead accumulation in the vegetative parts of Triticum aestivum L. Heliyon. 2020;6:e04188. https://doi.org/10.1016/j.heliyon.2020.e04188.
Checcucci A, Bazzicalupo M, Mengoni A. Exploiting nitrogen-fixing rhizobial symbionts genetic resources for improving phytoremediation of contaminated soils. In: Anjum N, Gill S, Tuteja N editor. Enhancing cleanup of environmental pollutants. Cham: Springer. 2017; p. 275-88. https://doi.org/10.1007/978-3-319-55426-6_13.
Halim MA, Rahman MM, Megharaj M, Naidu R. Cadmium immobilization in the rhizosphere and plant cellular detoxification: Role of plant-growth-promoting rhizobacteria as a sustainable solution. J Agric Food Chem. 2020;68:13497-529. https://doi.org/10.1021/acs.jafc.0c04579.
Abdelkrim S, Jebara SH, Saadani O et al. In situ effects of Lathyrus sativus- PGPR to remediate and restore quality and fertility of Pb and Cd polluted soils. Ecotoxicol Environ Saf. 2020;192:110260. https://doi.org/10.1016/j.ecoenv.2020.110260.
Garg N, Bhandari P, Kashyap L, Singh S. Silicon nutrition and arbuscular mycorrhizal fungi. In: Deshmukh R, Durgesh K. Tripathi GG editor. Metalloids in plants. USA: John Wiley and Sons. 2020; p. 315-54. https://doi.org/10.1002/9781119487210.ch16.
Mathew DC, Ho YN, Gicana RG, Mathew GM, Chien MC, Huang CC. A rhizosphere-associated symbiont, Photobacterium spp. strain MELD1 and its targeted synergistic activity for phytoprotection against mercury. Chauvat F editor. PLoS One. 2015;10:e0121178. https://doi.org/10.1371/journal.pone.0121178.
Ma Y, Rajkumar M, Zhang C, Freitas H. Inoculation of Brassica oxyrrhina with plant growth promoting bacteria for the improvement of heavy metal phytoremediation under drought conditions. J Hazard Mater. 2016;320:36-44. https://doi.org/10.1016/j.jhazmat.2016.08.009.
Funes Pinter I, Salomon MV, Berli F, Bottini R, Piccoli P. Characterization of the As(III) tolerance conferred by plant growth promoting rhizobacteria to in vitro-grown grapevine. Appl Soil Ecol. 2017;109:60-68. https://doi.org/10.1016/j.apsoil.2016.10.003.
Ma Y, Rajkumar M, Moreno A, Zhang C, Freitas H. Serpentine endophytic bacterium Pseudomonas azotoformans ASS1 accelerates phytoremediation of soil metals under drought stress. Chemosphere. 2017;185:75-85. https://doi.org/10.1016/j.chemosphere.2017.06.135.
Ali A, Guo D, Mahar A et al. Role of Streptomyces pactum in phytoremediation of trace elements by Brassica juncea in mine polluted soils. Ecotoxicol Environ Saf. 2017;144:387-95. https://doi.org/10.1016/j.ecoenv.2017.06.046.
Li Y, Liu K, Wang Y et al. Improvement of cadmium phytoremediation by Centella asiatica L. after soil inoculation with cadmium-resistant Enterobacter sp. FM-1. Chemosphere. 2018;202:280-88. https://doi.org/10.1016/j.chemosphere.2018.03.097.
Wang Q, Zhang WJ, He LY, Sheng XF. Increased biomass and quality and reduced heavy metal accumulation of edible tissues of vegetables in the presence of Cd-tolerant and immobilizing Bacillus megaterium H3. Ecotoxicol Environ Saf. 2018;148:269-74. https://doi.org/10.1016/j.ecoenv.2017.10.036.
Han H, Sheng X, Hu J, He L, Wang Q. Metal-immobilizing Serratia liquefaciens CL-1 and Bacillus thuringiensis X30 increase biomass and reduce heavy metal accumulation of radish under field conditions. Ecotoxicol Environ Saf. 2018;161:526-33. https://doi.org/10.1016/j.ecoenv.2018.06.033.
Dutta P, Karmakar A, Majumdar S, Roy S. Klebsiella pneumoniae (HR1) assisted alleviation of Cd(II) toxicity in Vigna mungo: a case study of biosorption of heavy metal by an endophytic bacterium coupled with plant growth promotion. Euro-Mediterranean J Environ Integr. 2018;3:27. https://doi.org/10.1007/s41207-018-0069-6.
Govarthanan M, Mythili R, Selvankumar T, Kamala-Kannan S, Kim H. Myco-phytoremediation of arsenic- and lead-contaminated soils by Helianthus annuus and wood rot fungi, Trichoderma sp. isolated from decayed wood. Ecotoxicol Environ Saf. 2018;151:279-84. https://doi.org/10.1016/j.ecoenv.2018.01.020.
Wiangkham N, Prapagdee B. Potential of Napier grass with cadmium-resistant bacterial inoculation on cadmium phytoremediation and its possibility to use as biomass fuel. Chemosphere. 2018;201:511-18. https://doi.org/10.1016/j.chemosphere.2018.03.039.
Ahemad M. Remediation of metalliferous soils through the heavy metal resistant plant growth promoting bacteria: Paradigms and prospects. Arab J Chem. 2019;12:1365-77. https://doi.org/10.1016/j.arabjc.2014.11.020.
Khanna K, Jamwal VL, Gandhi SG, Ohri P, Bhardwaj R. Metal resistant PGPR lowered Cd uptake and expression of metal transporter genes with improved growth and photosynthetic pigments in Lycopersicon esculentum under metal toxicity. Sci Rep. 2019;9:5855. https://doi.org/10.1038/s41598-019-41899-3.
Haruma T, Yamaji K, Ogawa K, Masuya H, Sekine Y, Kozai N. Root-endophytic Chaetomium cupreum chemically enhances aluminium tolerance in Miscanthus sinensis via increasing the aluminium detoxicants, chlorogenic acid and oosporein. Sarangi PK editor. PLoS One. 2019;14:e0212644. https://doi.org/10.1371/journal.pone.0212644.
Jin Z, Deng S, Wen Y et al. Application of Simplicillium chinense for Cd and Pb biosorption and enhancing heavy metal phytoremediation of soils. Sci Total Environ. 2019;697:134148. https://doi.org/10.1016/j.scitotenv.2019.134148.
Rathore SS, Shekhawat K, Dass A, Kandpal BK, Singh VK. Phytoremediation mechanism in Indian mustard (Brassica juncea) and its enhancement through agronomic interventions. Proc Natl Acad Sci India Sect B Biol Sci. 2019;89:419-27. https://doi.org/10.1007/s40011-017-0885-5.
Ma Y, Rajkumar M, Oliveira RS, Zhang C, Freitas H. Potential of plant beneficial bacteria and arbuscular mycorrhizal fungi in phytoremediation of metal-contaminated saline soils. J Hazard Mater. 2019;379:120813. https://doi.org/10.1016/j.jhazmat.2019.120813.
Hou J, Liu W, Wu L et al. Rhodococcus sp. NSX2 modulates the phytoremediation efficiency of a trace metal-contaminated soil by reshaping the rhizosphere microbiome. Appl Soil Ecol. 2019;133:62-69. https://doi.org/10.1016/j.apsoil.2018.09.009.
Ren CG, Kong CC, Wang SX, Xie ZH. Enhanced phytoremediation of uranium-contaminated soils by arbuscular mycorrhiza and rhizobium. Chemosphere. 2019;217:773-79. https://doi.org/10.1016/j.chemosphere.2018.11.085.
Wang L, Lin H, Dong Y, Li B, He Y. Effects of endophytes inoculation on rhizosphere and endosphere microecology of Indian mustard (Brassica juncea) grown in vanadium-contaminated soil and its enhancement on phytoremediation. Chemosphere. 2020;240:124891. https://doi.org/10.1016/j.chemosphere.2019.124891.
Rai PK, Kim KH, Lee SS, Lee JH. Molecular mechanisms in phytoremediation of environmental contaminants and prospects of engineered transgenic plants/microbes. Sci Total Environ. 2020;705:135858. https://doi.org/10.1016/j.scitotenv.2019.135858.
Wu Y, Ma L, Liu Q et al. The plant-growth promoting bacteria promote cadmium uptake by inducing a hormonal crosstalk and lateral root formation in a hyperaccumulator plant Sedum alfredii. J Hazard Mater. 2020;395:122661. https://doi.org/10.1016/j.jhazmat.2020.122661.
Jain D, Kour R, Bhojiya AA et al. Zinc tolerant plant growth promoting bacteria alleviates phytotoxic effects of zinc on maize through zinc immobilization. Sci Rep. 2020;10:13865. https://doi.org/10.1038/s41598-020-70846-w.
Sagonda T, Adil MF, Sehar S et al. Physio-ultrastructural footprints and iTRAQ-based proteomic approach unravel the role of Piriformospora indica colonization in counteracting cadmium toxicity in rice. Ecotoxicol Environ Saf. 2021;220:112390. https://doi.org/10.1016/j.ecoenv.2021.112390.
Han H, Zhang H, Qin S et al. Mechanisms of Enterobacter bugandensis TJ6 immobilization of heavy metals and inhibition of Cd and Pb uptake by wheat based on metabolomics and proteomics. Chemosphere. 2021;276:130157. https://doi.org/10.1016/j.chemosphere.2021.130157.
Qin S, Wu X, Han H, Pang F, Zhang J, Chen Z. Polyamine-producing bacterium Bacillus megaterium N3 reduced Cd accumulation in wheat and increased the expression of DNA repair- and plant hormone- related proteins in wheat roots. Environ Exp Bot. 2021;189:104563. https://doi.org/10.1016/j.envexpbot.2021.104563.
Photolo MM, Sitole L, Mavumengwana V, Tlou MG. Genomic and physiological investigation of heavy metal resistance from plant endophytic methylobacterium radiotolerans MAMP 4754, isolated from Combretum erythrophyllum. Int J Environ Res Public Health. 2021;18:997. https://doi.org/10.3390/ijerph18030997.
Fahsi N, Mahdi I, Mesfioui A, Biskri L, Allaoui A. Plant growth-promoting rhizobacteria isolated from the Jujube (Ziziphus lotus) plant enhance wheat growth, Zn uptake and heavy metal tolerance. Agriculture. 2021;11:316. https://doi.org/10.3390/agriculture11040316.
Li Q, Xing Y, Fu X et al. Biochemical mechanisms of rhizospheric Bacillus subtilis-facilitated phytoextraction by alfalfa under cadmium stress – Microbial diversity and metabolomics analyses. Ecotoxicol Environ Saf. 2021;212:112016. https://doi.org/10.1016/j.ecoenv.2021.112016.
Aktar W, Sengupta D, Chowdhury A. Impact of pesticides use in agriculture: their benefits and hazards. Interdiscip Toxicol. 2009;2:1-12. https://doi.org/10.2478/v10102-009-0001-7.
Jin S, Bluemling B, Mol APJ. Mitigating land pollution through pesticide packages – The case of a collection scheme in Rural China. Sci Total Environ. 2018;622-23:502-09. https://doi.org/10.1016/j.scitotenv.2017.11.330.
Sánchez-Bayo F. Indirect effect of pesticides on insects and other arthropods. Toxics. 2021;9:177. https://doi.org/10.3390/toxics9080177.
Giménez–Moolhuyzen M, van der Blom J, Lorenzo–Mínguez P, Cabello T, Crisol–Martínez E. Photosynthesis inhibiting effects of pesticides on sweet pepper leaves. Insects. 2020;11:69. https://doi.org/10.3390/insects11020069.
Sharma A, Kumar V, Kumar R, Shahzad B, Thukral AK, Bhardwaj R. Brassinosteroid-mediated pesticide detoxification in plants: A mini-review. Tejada Moral M editor. Cogent Food Agric. 2018;4:1436212. https://doi.org/10.1080/23311932.2018.1436212.
Prasad SM, Zeeshan M, Kumar D. Toxicity of endosulfan on growth, photosynthesis and nitrogenase activity in two species of Nostoc (Nostoc muscorum and Nostoc calcicola). Toxicol Environ Chem. 2011;93:513-25. https://doi.org/10.1080/02772248.2010.542157.
Fernandes C, Figueira E, Tauler R, Bedia C. Exposure to chlorpyrifos induces morphometric, biochemical and lipidomic alterations in green beans (Phaseolus vulgaris). Ecotoxicol Environ Saf. 2018;156:25-33. https://doi.org/10.1016/j.ecoenv.2018.03.005.
Jena S, Acharya S, Mohapatra PK. Variation in effects of four OP insecticides on photosynthetic pigment fluorescence of Chlorella vulgaris Beij. Ecotoxicol Environ Saf. 2012;80:111-17. https://doi.org/10.1016/j.ecoenv.2012.02.016.
Grosicka-Maci?g E. Biologiczne skutki stresu oksydacyjnego wywo?anego dzia?aniem pestycydów. Postepy Hig Med Dosw. 2011;65:357-66. https://doi.org/10.5604/17322693.948816.
Hatamleh AA, Danish M, Al-Dosary MA, El-Zaidy M, Ali S. Physiological and oxidative stress responses of Solanum lycopersicum (L.) (tomato) when exposed to different chemical pesticides. RSC Adv. 2022;12:7237-52. https://doi.org/10.1039/D1RA09440H.
Sule RO, Condon L, Gomes AV. A common feature of pesticides: Oxidative stress—The role of oxidative stress in pesticide-induced toxicity. Zhou X editor. Oxid Med Cell Longev. 2022;2022:1-31. https://doi.org/10.1155/2022/5563759.
Srivastava AK, Singh D. Assessment of malathion toxicity on cytophysiological activity, DNA damage and antioxidant enzymes in root of Allium cepa model. Sci Rep. 2020;10:886. https://doi.org/10.1038/s41598-020-57840-y.
Yengkokpam P, Mazumder PB. Antioxidant enzymatic activities and profiling of gene expression associated with organophosphate stress tolerance in Solanum melongena L.cv. Longai. 3 Biotech. 2021;11:510. https://doi.org/10.1007/s13205-021-03061-7.
Wang P, Yang X, Huang WW et al. Effect of pesticide 1-[6-chloro-3-methyl-pyridyl-8-nitro-7-methyl-1 2 3 5 6 7-hexahydro imidazo (1,2a)]-pyridine when responding to a wheat plant’s antioxidant defense system. Food Chem. 2014;146:569-76. https://doi.org/10.1016/j.foodchem.2013.09.085.
Shakir SK, Irfan S, Akhtar B et al. Pesticide-induced oxidative stress and antioxidant responses in tomato (Solanum lycopersicum) seedlings. Ecotoxicology. 2018;27:919-35. https://doi.org/10.1007/s10646-018-1916-6.
Yan A, Wang Y, Tan SN, Mohd Yusof ML, Ghosh S, Chen Z. Phytoremediation: A promising approach for revegetation of heavy metal-polluted land. Front Plant Sci. 2020;11. https://doi.org/10.3389/fpls.2020.00359.
Doty SL. Enhancing phytoremediation through the use of transgenics and endophytes. New Phytol. 2008;179:318-33. https://doi.org/10.1111/j.1469-8137.2008.02446.x.
Rodríguez-Llorente ID, Lafuente A, Doukkali B, Caviedes MA, Pajuelo E. Engineering copper hyper accumulation in plants by expressing a prokaryotic copC gene. Environ Sci Technol. 2012;46:12088-097. https://doi.org/10.1021/es300842s.
Pérez-Palacios P, Agostini E, Ibáñez SG et al. Removal of copper from aqueous solutions by rhizofiltration using genetically modified hairy roots expressing a bacterial Cu-binding protein. Environ Technol. 2017;38:2877-88. https://doi.org/10.1080/09593330.2017.1281350.
Yu P, Yuan J, Deng X, Ma M, Zhang H. Subcellular targeting of bacterial CusF enhances Cu accumulation and alters root to shoot Cu translocation in Arabidopsis. Plant Cell Physiol. 2014;55:1568-81. https://doi.org/10.1093/pcp/pcu087.
Liu D, An Z, Mao Z, Ma L, Lu Z. Enhanced heavy metal tolerance and accumulation by transgenic sugar beets expressing Streptococcus thermophilus StGCS-GS in the presence of Cd, Zn and Cu alone or in combination. Wu K editor. PLoS One. 2015;10:e0128824. https://doi.org/10.1371/journal.pone.0128824.
Shim D, Kim S, Choi YI et al. Transgenic poplar trees expressing yeast cadmium factor 1 exhibit the characteristics necessary for the phytoremediation of mine tailing soil. Chemosphere. 2013;90:1478-86. https://doi.org/10.1016/j.chemosphere.2012.09.044.
Bizily SP, Rugh CL, Summers AO, Meagher RB. Phytoremediation of methylmercury pollution: merB expression in Arabidopsis thaliana confers resistance to organomercurials. Proc Natl Acad Sci. 1999;96:6808-13. https://doi.org/10.1073/pnas.96.12.6808.
Nagata T, Morita H, Akizawa T, Pan-Hou H. Development of a transgenic tobacco plant for phytoremediation of methylmercury pollution. Appl Microbiol Biotechnol. 2010;87:781-86. https://doi.org/10.1007/s00253-010-2572-9.
Kiyono M, Oka Y, Sone Y et al. Expression of the bacterial heavy metal transporter MerC fused with a plant SNARE, SYP121, in Arabidopsis thaliana increases cadmium accumulation and tolerance. Planta. 2012;235:841-50. https://doi.org/10.1007/s00425-011-1543-4.
Sasaki Y, Hayakawa T, Inoue C, Miyazaki A, Silver S, Kusano T. Generation of mercury-hyper accumulating plants through transgenic expression of the bacterial mercury membrane transport protein MerC. Transgenic Res. 2006;15:615-25. https://doi.org/10.1007/s11248-006-9008-4.
Xu S, Sun B, Wang R et al. Overexpression of a bacterial mercury transporter MerT in Arabidopsis enhances mercury tolerance. Biochem Biophys Res Commun. 2017;490:528-34. https://doi.org/10.1016/j.bbrc.2017.06.073.
Nagata T, Nakamura A, Akizawa T, Pan-Hou H. Genetic engineering of transgenic tobacco for enhanced uptake and bioaccumulation of mercury. Biol Pharm Bull. 2009;32:1491-95. https://doi.org/10.1248/bpb.32.1491.
Peng RH, Fu XY, Zhao W et al. Phytoremediation of phenanthrene by transgenic plants transformed with a naphthalene dioxygenase system from Pseudomonas. Environ Sci Technol. 2014;48:12824-32. https://doi.org/10.1021/es5015357.
Dixit P, Mukherjee PK, Sherkhane PD, Kale SP, Eapen S. Enhanced tolerance and remediation of anthracene by transgenic tobacco plants expressing a fungal glutathione transferase gene. J Hazard Mater. May 2011. https://doi.org/10.1016/j.jhazmat.2011.05.018.
Stepanova AY, Orlova EV, Teteshonok DV, Dolgikh YI. Obtaining transgenic alfalfa plants for improved phytoremediation of petroleum-contaminated soils. Russ J Genet Appl Res. 2016;6:705-11. https://doi.org/10.1134/S2079059716060083.
Shimizu M, Kimura T, Koyama T et al. Molecular breeding of transgenic rice plants expressing a bacterial chlorocatechol dioxygenase gene. Appl Environ Microbiol. 2002;68:4061-66. https://doi.org/10.1128/AEM.68.8.4061-4066.2002.
Du H, Shen X, Huang Y, Huang M, Zhang Z. Over expression of vitreoscilla heamoglobin increases waterlogging tolerance in Arabidopsis and maize. BMC Plant Biol. 2016;16:35. https://doi.org/10.1186/s12870-016-0728-1.
Amiri RM, Yur’eva NO, Shimshilashvili KR et al. Expression of acyl?lipid ?12?desaturase gene in prokaryotic and eukaryotic cells and its effect on cold stress tolerance of potato. J Integr Plant Biol. 2010;52:289-97. https://doi.org/10.1111/j.1744-7909.2010.00890.x.
Yu TF, Xu ZS, Guo JK et al. Improved drought tolerance in wheat plants overexpressing a synthetic bacterial cold shock protein gene SeCspA. Sci Rep. 2017;7:44050. https://doi.org/10.1038/srep44050.
Joo J, Choi HJ, Lee YH et al. Over-expression of BvMTSH, a fusion gene for maltooligosyltrehalose synthase and maltooligosyltrehalose trehalohydrolase, enhances drought tolerance in transgenic rice. BMB Rep. 2014;47:27-32. https://doi.org/10.5483/BMBRep.2014.47.1.064.
Goel D, Singh AK, Yadav V, Babbar SB, Murata N, Bansal KC. Transformation of tomato with a bacterial codA gene enhances tolerance to salt and water stresses. J Plant Physiol. 2011;168:1286-94. https://doi.org/10.1016/j.jplph.2011.01.010.
Tran N-HT, Oguchi T, Matsunaga E, Kawaoka A, Watanabe KN, Kikuchi A. Transcriptional enhancement of a bacterial choline oxidase A gene by an HSP terminator improves the glycine betaine production and salinity stress tolerance of Eucalyptus camaldulensis trees. Plant Biotechnol. 2018;35:215-24. https://doi.org/10.5511/plantbiotechnology.18.0510b.
Wei D, Zhang W, Wang C et al. Genetic engineering of the biosynthesis of glycinebetaine leads to alleviate salt-induced potassium efflux and enhances salt tolerance in tomato plants. Plant Sci. 2017;257:74-83. https://doi.org/10.1016/j.plantsci.2017.01.012.
Cho JI, Lim HM, Siddiqui ZS et al. Over-expression of PsGPD, a mushroom glyceraldehyde-3-phosphate dehydrogenase gene, enhances salt tolerance in rice plants. Biotechnol Lett. 2014;36:1641-48. https://doi.org/10.1007/s10529-014-1522-5.
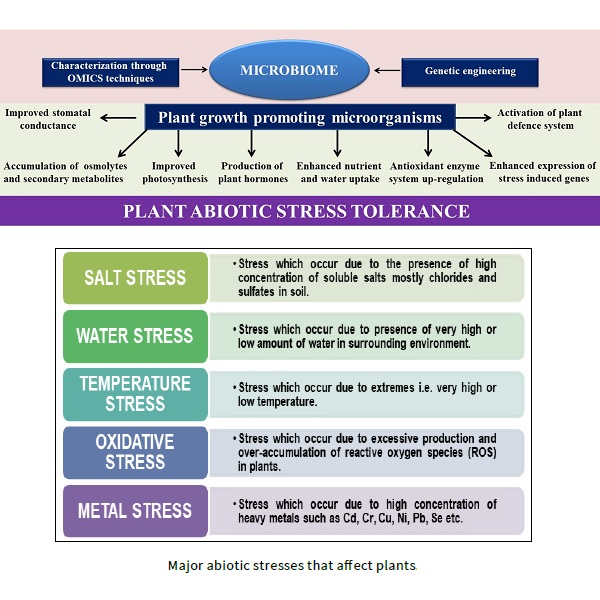
Downloads
Published
Versions
- 01-07-2023 (2)
- 13-05-2023 (1)
How to Cite
Issue
Section
License
Copyright (c) 2022 Renu Soni, Geeta Prakash, Shweta Sharma, Dwaipayan Sinha, Reema Mishra

This work is licensed under a Creative Commons Attribution 4.0 International License.
Copyright and Licence details of published articles
Authors who publish with this journal agree to the following terms:
- Authors retain copyright and grant the journal right of first publication with the work simultaneously licensed under a Creative Commons Attribution License that allows others to share the work with an acknowledgement of the work's authorship and initial publication in this journal.
- Authors are able to enter into separate, additional contractual arrangements for the non-exclusive distribution of the journal's published version of the work (e.g., post it to an institutional repository or publish it in a book), with an acknowledgement of its initial publication in this journal.
Open Access Policy
Plant Science Today is an open access journal. There is no registration required to read any article. All published articles are distributed under the terms of the Creative Commons Attribution License (CC Attribution 4.0), which permits unrestricted use, distribution, and reproduction in any medium, provided the original author and source are credited (https://creativecommons.org/licenses/by/4.0/). Authors are permitted and encouraged to post their work online (e.g., in institutional repositories or on their website) prior to and during the submission process, as it can lead to productive exchanges, as well as earlier and greater citation of published work (See The Effect of Open Access).