Variation of the chemical and biochemical responses to salinity during germination and early growth of seedlings of two populations of Agave durangensis Gentry
DOI:
https://doi.org/10.14719/pst.2963Keywords:
Maguey cenizo, anthocyanin accumulation, antioxidant enzymes, salt tolerance, seed provenanceAbstract
Agave durangensis (Asparagaceae) sustains a mescal industry in Mexico. The main reproductive strategy of the species is by seeds. The increased demand for agave-based beverages encourages producers to seek new cultivation areas. However, more than half of the territory of the country includes arid and semiarid zones, which are highly affected by salinity. The aim of the current study was to determine if salinity triggers different seed germination potential and variable biochemical and chemical responses in seedlings of two populations of A. durangensis, that might confer different tolerance to salinity. Seeds from each population were irrigated with four salinity treatments. Germination potential, as well as growth parameters and biochemical and chemical attributes of seedlings, were determined. Although with reduced germinability and germination speed, seeds of the two populations were able to germinate even under the strongest NaCl concentration (100 mM) evaluated. Effects in the growth parameters were registered; however, the seedlings of both populations survived throughout the experiments, increasing chlorophyll content and cell viability in most saline treatments. The enzymatic defense mechanism and the accumulation of proline were activated in a salt-dependent manner, which did not occur with the phenolic compounds; however, monomeric anthocyanin accumulation was outstanding under the two strongest NaCl concentrations evaluated. Important interpopulation differences were registered in each type of response, which were differently regulated under variable NaCl concentrations, some of them being more important than others under a particular saline condition. Seeds from the population Durango were more sensitive to salinity.
Downloads
References
Acosta-Motos JR, Ortuño MF, Bernal-Vicente A, Díaz-Vivancos P, Sánchez-Blanco MJ, Hernández JA. Plant responses to salt stress: Adaptive mechanisms. Agronomy. 2017; 7:18. https://doi.org/10.3390/agronomy7010018
Farooq F, Rashid N, Ibrar D, Hasnain Z, Ullah R, Nawaz M, et al. Impact of varying levels of soil salinity on emergence, growth and biochemical attributes of four Moringa oleifera landraces. PLoS ONE. 2022; 17(2):e0263978. https://doi.org/10.1371/journal.pone.0263978
Arif Y, Singh P, Siddiqui H, Bajguz A, Hayat S. Salinity induced physiological and biochemical changes in plants: An omic approach towards salt stress tolerance. Plant Physiol Biochem. 2020; 156:64-77. https://doi.org/10.1016/j.plaphy.2020.08.042
Oueslati S, Karray-Bouraoui N, Attia H, Rabhi M, Ksouri R, Lachaal M. Physiological and antioxidant responses of Mentha pulegium (Pennyroyal) to salt stress. Acta Physiol Plant. 2010; 32(2):289-96. https://doi.org/10.1007/s11738-009-0406-0
Gharsallah C, Fakhfakh H, Grubb D, Gorsane F. Effect of salt stress on ion concentration, proline content, antioxidant enzyme activities and gene expression in tomato cultivars. AoB PLANTS. 2016; 8:plw055 https://doi.org/10.1093/aobpla/plw055
Ahmad P, Jaleel CA, Salem MA, Nabi G, Sharma S. Roles of enzymatic and nonenzymatic antioxidants in plants during abiotic stress. Crit Rev Biotechnol. 2010;30(3):161-75. https://doi.org/10.3109/07388550903524243
Almaraz-Abarca N, Delgado-Alvarado EA, Torres-Morán MI, Herrera-Corral J, Ávila-Reyes JA, Naranjo-Jiménez N, Uribe-Soto JN. Genetic variability in natural populations of Agave durangensis (Agavaceae) revealed by morphological and molecular traits. Southwest Nat. 2013; 58(3):314–24. https://doi.org/10.1894/0038-4909-58.3.314
Perez-Aguilar LY, Plata-Rocha W, Monjardin-Armenta SA, Franco-Ochoa C, Zambrano-Medina YG. The identification and classification of arid zones through multicriteria evaluation and geographic information systems—Case study: Arid regions of northwest Mexico. ISPRS Int J GeoInf. 2021;10(11):720. https://doi.org/10.3390/ijgi10110720
Nobel PS, Berry WL. Element responses of Agaves. Amer J Bot. 1985; 72(5):686-94. https://doi.org/10.2307/2443680
Schuch UK, Kelly JJ. Salinity tolerance of cacti and succulents. Turfgrass, Landscape and Urban IPM Research Summary. 2008; 55:61-66. http://hdl.handle.net/10150/216639
El-Bagoury OH, El-Agroudy MH, Shenouda MA. Effect of salinity levels on growth of six plant species. Egypt J Agron. 1993;18(1/2):129-143
Miyamoto S. Salt tolerance of landscape plants common to the Southwest. El Paso: Texas Water Resources Institute. 2008
Bergsten SJ, Koeser AK, Stewart JR. Evaluation of the impacts of salinity on biomass and nutrient levels of Agave species with agricultural potential in semiarid regions. HortScience 2016;51(1):30-35. https://doi.org/10.21273/hortsci.51.1.30
Good-Ávila SV, Souza V, Gaut VS, Eguiarte LE. Timing and rate of speciation in Agave (Agavaceae). Proc Natl Acad Sci USA. 2006;103(24):9124–29. https://doi.org/10.1073/pnas.0603312103
Lini? I, Šamec D, Grúz J, Bok VV, Strnad M, Salopek-Sondi B. Involvement of phenolic acids in short-term adaptation to salinity stress is species-specific among Brassicaceae. Plants. 2019; 8(6):155. https://doi.org/10.3390/plants8060155
Kumar S, Li G, Yang J, Huang X, Ji Q, Zhou K, et al. Investigation of an antioxidative system for salinity tolerance in Oenanthe javanica. Antioxidants. 2020; 9(10):940. https://doi.org/10.3390/antiox9100940
Gengmao Z, Yu H, Xing S, Shihui L, Quanmei S, Changhai W. Salinity stress increases secondary metabolites and enzyme activity in safflower. Ind Crops Prod. 2015; 64(1):175-181. https://doi.org/10.1016/j.indcrop.2014.10.058
Elavarthi S, Martin B. Spectrophotometric assays for antioxidant enzymes in plants. In: Sunkar R, editor. Plant stress tolerance. Methods in Molecular Biology 639. New York: Humana Press; 2010; 273-280. https://doi.org/10.1007/978-1-60761-702-0_16
Vasavilbazo-Saucedo A, Almaraz-Abarca N, González-Ocampo HA, Ávila-Reyes JA, González-Valdez LS, Luna-González A, et al. Phytochemical characterization and antioxidant properties of the wild edible acerola Malpighia umbellata Rose. CYTA - J Food. 2018; 16(1):698-706. https://doi.org/10.1080/19476337.2018.1475424
Ordoñez AAL, Gomez JD, Vattuone MA, lsla MI. Antioxidant activities of Sechium edule (Jacq.) Swartz extracts. Food Chem. 2006; 97(3):452-58. https://doi.org/10.1016/j.foodchem.2005.05.024
Herald TJ, Gadgil P, Perumal R, Bean SR, Wilson JD. High?throughput micro?plate HCl–vanillin assay for screening tannin content in sorghum grain. Journal of the Science of Food and Agriculture. 2014; 94(10): 2133-36. https://doi.org/10.1002/jsfa.6538
So V, Pocasap P, Sutthanut K, Sethabouppha B, Thukhammee W, Wattanathorn J, et al. Effect of harvest age on total phenolic, total anthocyanin content, bioactive antioxidant capacity and antiproliferation of black and white glutinous rice sprouts. Appl Sci. 2020; 10(20):7051. https://doi.org/10.3390/app10207051
Chavan RR, Bhinge SD, Bhutkar MA, Randive DS, Wadkar GH, Todkar SS, et al. Characterization, antioxidant, antimicrobial and cytotoxic activities of green synthesized silver and iron nanoparticles using alcoholic Blumea eriantha DC plant extract. Mater Today Commun. 2020; 24:101320. https://doi.org/10.1016/j.mtcomm.2020.101320
Vyas D, Kumar S. Tea (Camellia sinensis (L.) O. Kuntze) clone with lower period of winter dormancy exhibits lesser cellular damage in response to low temperature. Plant Physiology and Biochemistry. 2005; 43(4):383-88. https://doi.org/ 10.1016/j.plaphy.2005.02.016
Wa?kiewicz A, Muzolf-Panek M, Goli?ski P. Phenolic content changes in plants under salt stress. In: Ahmad P, Azooz MM, Prasad MNV, editors. Ecophysiology and responses of plants under salt stress. New York: Springer; 2013;283-314. https://doi.org/10.1007/978-1-4614-4747-4
Wallander-Compean L, Almaraz-Abarca N, Alejandre-Iturbide G, Uribe-Soto JN, Ávila-Reyes JA, Torres-Ricario R, et al. Variación fenológica y morfométrica de Phaseolus vulgaris (Fabaceae) de cinco poblaciones silvestres de Durango, México. Bot Sci. 2022; 100(3):563-78. https://doi.org/10.17129/botsci.2981
Dehnavi AR, Zahedi M, Ludwiczak A, Perez SC, Piernik A. Effect of salinity on seed germination and seedling development of sorghum (Sorghum bicolor (L.) Moench) genotypes. Agronomy. 2020; 10(6):859. https://doi.org/10.3390/agronomy10060859
Hernández-Pacheco CE, Almaraz-Abarca N, Rojas-López M, Torres-Ricario R, Ávila-Reyes JA, González-Valdez LS. et al. Salinity generates varying chemical and biochemical responses in Physalis ixocarpa (Solanaceae) during different times of exposure. Electron J Biotechnol. 2022; 59: 25-35. https://doi.org/10.1016/j.ejbt.2022.06.002
Uçarli C. Effects of salinity on seed germination and early seedling stage. In: Fahad S, Saud S, Chen Y, Wu C, Wang D, editors. Abiotic stress in plants. IntechOpen; 2021. https://doi.org/10.5772/intechopen.93647
Aazami MA, Rasouli F, Ebrahimzadeh A. Oxidative damage, antioxidant mechanism and gene expression in tomato responding to salinity stress under in vitro conditions and application of iron and zinc oxide nanoparticles on callus induction and plant regeneration. BMC Plant Biol. 2021; 21:597. https://doi.org/10.1186/s12870-021-03379-7
Rahman M, Soomro UA, Haq MZ, Gul S. Effects of NaCl salinity on wheat (Triticum aestivum L.) cultivars. World J Agric Sci. 2008; 4(3):398–403
Barriada-Bernal G, Almaraz-Abarca N, Gallardo-Velázquez T, Torres-Morán I, Herrera-Arrieta Y, González-Elizondo S, et al. Seed vigor variation of Agave durangensis Gentry (Agavaceae). Am J Plant Sci. 2013;4(11):2227-39. https://doi.org/10.4236/ajps.2013.411276
Ji X, Tang J, Zhang J. Effects of salt stress on the morphology, growth and physiological parameters of Juglans microcarpa L. seedlings. Plants. 2022;11(18): 2381. https://doi.org/10.3390/plants11182381
Guo X, Ahmad N, Zhao S, Zhao C, Zhong W, Wang X, et al. Effect of salt stress on growth and physiological properties of Asparagus seedlings. Plants. 2022;1(21):2836. https://doi.org/10.3390/plants11212836
Farooq M, Ahmad R, Shahzad M, Sajjad Y, Hassam A, Shah MM, et al. Differential variations in total flavonoid content and antioxidant enzymes activities in pea under different salt and drought stresses. Sci Hortic. 2021;287:110258. https://doi.org/10.1016/j.scienta.2021.110258
Bilska K, Wojciechowska N, Alipour S, Kalemba EM. Ascorbic acid—The little-known antioxidant in woody plants. Antioxidants. 2019;8:645. https://doi.org/10.3390/antiox8120645
Naing AH, CK Kim. Abiotic stress-induced anthocyanins in plants: Their role in tolerance to abiotic stresses. Physiol Plant 2021; 172:1711–23. https://doi.org/10.1111/ppl.13373
Azeem M, Pirjan K, Qasim M, Mahmood A, Javed T, Muhammad H, et al. Salinity stress improves antioxidant potential by modulating physio-biochemical responses in Moringa oleifera Lam. Sci Rep. 2023; 13(1):1-17. https://doi.org/10.1038/s41598-023-29954-6
Sevengor S, Yasar F, Kusvuran S, Ellialtioglu S. The effect of salt stress on growth chlorophyll content, lipid peroxidation and antioxidative enzymes of pumpkin seedlings. Afr J Agric Res. 2011; 6:4920-24
Yamagata K. Carotenoids regulate endothelial functions and reduce the risk of cardiovascular disease. In: Cvetkavic D, Nikolic G, editors. Carotenoids. London: IntechOpen; 2017; 105-121. https://doi.org/10.5772/65523
Mirkovic T, Ostroumov EE, Anna JM, van Grondelle R, Govindjee R, Scholes GD. Light absorption and energy transfer in the antenna complexes of photosynthetic organisms. Chem Rev. 2017; 117(2):249–93
Minh LT, Khang DT, Ha PTT, Tuyen PT, Minh TN, Quan NV, et al. Effects of salinity stress on growth and phenolics of Rice (Oryza sativa L.). International Letters of Natural Sciences 2016; 57:1-10. https://doi.org/10.18052/www.scipress.com/ILNS.57.1
Xu Z, Mahmood K, Rothstein SJ. ROS induces anthocyanin production via late biosynthetic genes and anthocyanin deficiency confers the hypersensitivity to ROS-generating stresses in Arabidopsis. Plant and Cell Physiology. 2017; 58(8):1364–77. https://doi.org/10.1093/pcp/pcx073
Khoo HE, Azlan A, Tang ST, Lim SM. Anthocyanidins and anthocyanins: Colored pigments as food, pharmaceutical ingredients, and the potential health benefits. Food Nutr Res. 2017; 61:1361779. https://doi.org/10.1080/16546628.2017.1361779
Chang WC, Chen MH, Lee TM. 2,3,5-triphenyltetrazolium reduction in the viability assay of Ulva fasciata (Chlorophyta) in response to salinity stress. Bot Bull Acad Sinica. 1999; 40(3):207-12
Tanaka J, Kiyoshi K, Kadokura T, Suzuki Kichiro, Nakayama S. Elucidation of the enzyme involved in 2,3,5-triphenyl tetrazolium chloride (TTC) staining activity and the relationship between TTC staining activity and fermentation profiles in Saccharomyces cerevisiae. J Biosci Bioeng. 2021; 131(4):396-404. https://doi.org/10.1016/j.jbiosc.2020.12.001
Ghazanfar B, Cheng Z, Ahmad I, Khan AR, Hanqiang L, Haiyan D, et al. Synergistic and individual effect of Glomus etunicatum root colonization and acetylsalicylic acid on root activity and architecture of tomato plants under moderate NaCl stress. Pak J Bot. 2015; 47(6):2047-54
Lu I-F, Sung M-S, Lee T-M. Salinity stress and hydrogen peroxide regulation of antioxidant defense system in Ulva fasciata. Mar Biol. 2006; 150:1-15. https://doi.org/10.1007/s00227-006-0323-3
Jacoby RP, Taylor NL, Millar AH. The role of mitochondrial respiration in salinity tolerance. Trends Plant Sci. 2011; 16(11):614-23. https://doi.org/10.1016/j.tplants.2011.08.002
Liu J, He Z. Small DNA methylation, big player in plant abiotic stress responses and memory. Front Plant Sci. 2020; 11:595603. https://doi.org/10.3389/fpls.2020.595603
Meltzer PS, Kallioniemi A, Trent JM. Chromosome alterations in human solid tumors. In: Vogelstein B, Kinzler KW, editors. The genetic basis of human cancer. New York: McGraw-Hill; 2002. p. 93-113
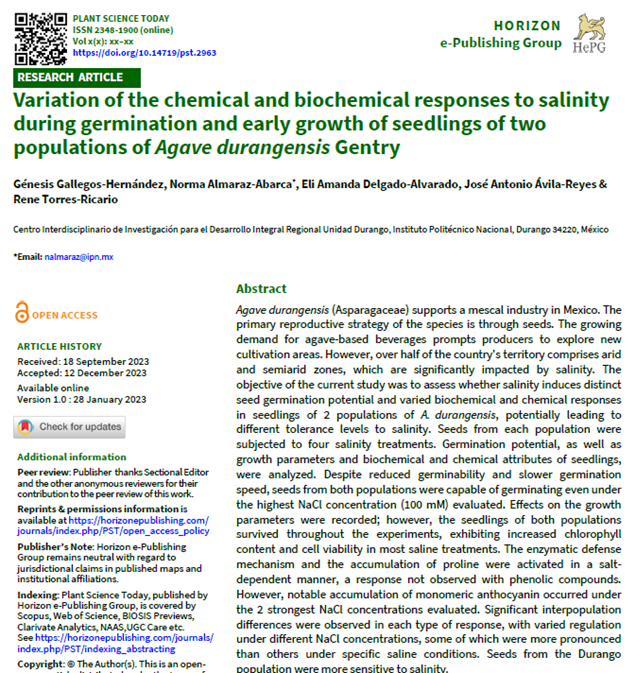
Downloads
Published
Versions
- 01-04-2024 (2)
- 28-01-2024 (1)
How to Cite
Issue
Section
License
Copyright (c) 2022 Génesis Gallegos-Hernández, Norma Almaraz-Abarca, Elí Amanda Delgado-Alvarado, José Antonio Ávila-Reyes, Rene Torres-Ricario

This work is licensed under a Creative Commons Attribution 4.0 International License.
Copyright and Licence details of published articles
Authors who publish with this journal agree to the following terms:
- Authors retain copyright and grant the journal right of first publication with the work simultaneously licensed under a Creative Commons Attribution License that allows others to share the work with an acknowledgement of the work's authorship and initial publication in this journal.
- Authors are able to enter into separate, additional contractual arrangements for the non-exclusive distribution of the journal's published version of the work (e.g., post it to an institutional repository or publish it in a book), with an acknowledgement of its initial publication in this journal.
Open Access Policy
Plant Science Today is an open access journal. There is no registration required to read any article. All published articles are distributed under the terms of the Creative Commons Attribution License (CC Attribution 4.0), which permits unrestricted use, distribution, and reproduction in any medium, provided the original author and source are credited (https://creativecommons.org/licenses/by/4.0/). Authors are permitted and encouraged to post their work online (e.g., in institutional repositories or on their website) prior to and during the submission process, as it can lead to productive exchanges, as well as earlier and greater citation of published work (See The Effect of Open Access).